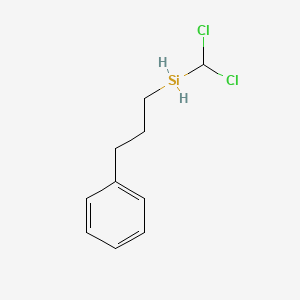
Dichloromethyl(3-phenylpropyl)silane
- Click on QUICK INQUIRY to receive a quote from our team of experts.
- With the quality product at a COMPETITIVE price, you can focus more on your research.
Overview
Description
Dichloromethyl(3-phenylpropyl)silane: is an organosilicon compound characterized by the presence of a silicon atom bonded to a dichloromethyl group and a 3-phenylpropyl group
Preparation Methods
Synthetic Routes and Reaction Conditions: The synthesis of Dichloromethyl(3-phenylpropyl)silane typically involves the reaction of 3-phenylpropylmagnesium bromide with dichloromethylchlorosilane. The reaction is carried out under anhydrous conditions to prevent hydrolysis of the chlorosilane. The general reaction scheme is as follows:
3-phenylpropylmagnesium bromide+dichloromethylchlorosilane→this compound+MgBrCl
Industrial Production Methods: Industrial production of this compound may involve similar synthetic routes but on a larger scale. The reaction conditions are optimized to ensure high yield and purity of the product. The use of continuous flow reactors and advanced purification techniques such as distillation and chromatography are common in industrial settings.
Chemical Reactions Analysis
Types of Reactions:
Substitution Reactions: Dichloromethyl(3-phenylpropyl)silane can undergo nucleophilic substitution reactions where the chlorine atoms are replaced by other nucleophiles such as alkoxides or amines.
Hydrosilylation: This compound can participate in hydrosilylation reactions, where it adds across double bonds in the presence of a catalyst, forming new silicon-carbon bonds.
Oxidation: The silicon-carbon bonds in this compound can be oxidized to form silanols or siloxanes.
Common Reagents and Conditions:
Substitution Reactions: Common reagents include sodium alkoxides, amines, and other nucleophiles. Reactions are typically carried out in polar aprotic solvents such as tetrahydrofuran (THF) or dimethylformamide (DMF).
Hydrosilylation: Catalysts such as platinum or rhodium complexes are used. The reaction is usually conducted at elevated temperatures.
Oxidation: Oxidizing agents such as hydrogen peroxide or peracids are used under mild conditions.
Major Products:
Substitution Reactions: Products include alkoxysilanes, aminosilanes, and other substituted silanes.
Hydrosilylation: The major products are organosilicon compounds with new silicon-carbon bonds.
Oxidation: Products include silanols and siloxanes.
Scientific Research Applications
Chemistry: Dichloromethyl(3-phenylpropyl)silane is used as a precursor in the synthesis of various organosilicon compounds. It is also employed in the development of new materials with unique properties, such as hydrophobic coatings and silicone-based polymers.
Biology: In biological research, this compound is used to modify surfaces of biomaterials to enhance their biocompatibility. It is also investigated for its potential use in drug delivery systems due to its ability to form stable bonds with organic molecules.
Medicine: this compound is explored for its potential in medical applications, including the development of silicone-based implants and prosthetics. Its ability to form biocompatible surfaces makes it a valuable material in medical device manufacturing.
Industry: In the industrial sector, this compound is used in the production of silicone-based adhesives, sealants, and coatings. Its unique chemical properties make it suitable for applications requiring high thermal stability and resistance to chemical degradation.
Mechanism of Action
The mechanism of action of Dichloromethyl(3-phenylpropyl)silane involves the formation of stable silicon-carbon bonds. The silicon atom in the compound can form strong covalent bonds with various organic and inorganic molecules, making it a versatile reagent in chemical synthesis. The molecular targets and pathways involved depend on the specific application and the nature of the reacting species.
Comparison with Similar Compounds
Dichloromethylsilane: Similar in structure but lacks the 3-phenylpropyl group.
Chloromethylsilane: Contains one chlorine atom and one methyl group bonded to silicon.
Trichlorosilane: Contains three chlorine atoms bonded to silicon.
Comparison:
- The 3-phenylpropyl group enhances the compound’s ability to interact with organic molecules, making it more suitable for applications in organic synthesis and materials science.
- Compared to Dichloromethylsilane and Trichlorosilane, this compound offers improved stability and reactivity, making it a valuable compound in various scientific and industrial applications.
Dichloromethyl(3-phenylpropyl)silane: is unique due to the presence of the 3-phenylpropyl group, which imparts distinct chemical properties and reactivity compared to other chlorosilanes.
Properties
Molecular Formula |
C10H14Cl2Si |
---|---|
Molecular Weight |
233.21 g/mol |
IUPAC Name |
dichloromethyl(3-phenylpropyl)silane |
InChI |
InChI=1S/C10H14Cl2Si/c11-10(12)13-8-4-7-9-5-2-1-3-6-9/h1-3,5-6,10H,4,7-8,13H2 |
InChI Key |
NVOADJUXMHJFLQ-UHFFFAOYSA-N |
Canonical SMILES |
C1=CC=C(C=C1)CCC[SiH2]C(Cl)Cl |
Origin of Product |
United States |
Disclaimer and Information on In-Vitro Research Products
Please be aware that all articles and product information presented on BenchChem are intended solely for informational purposes. The products available for purchase on BenchChem are specifically designed for in-vitro studies, which are conducted outside of living organisms. In-vitro studies, derived from the Latin term "in glass," involve experiments performed in controlled laboratory settings using cells or tissues. It is important to note that these products are not categorized as medicines or drugs, and they have not received approval from the FDA for the prevention, treatment, or cure of any medical condition, ailment, or disease. We must emphasize that any form of bodily introduction of these products into humans or animals is strictly prohibited by law. It is essential to adhere to these guidelines to ensure compliance with legal and ethical standards in research and experimentation.