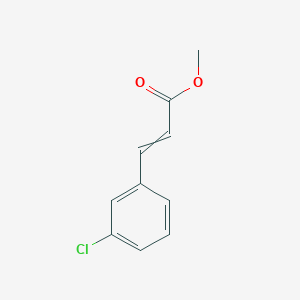
Methyl (E)-3-(3-chlorophenyl)acrylate
Overview
Description
Methyl (E)-3-(3-chlorophenyl)acrylate is an α,β-unsaturated ester characterized by a chlorine substituent at the meta position of the phenyl ring. Its synthesis typically involves palladium-catalyzed cross-coupling or direct C–H functionalization strategies. For example, it has been prepared via aerobic C–H activation using Pd(OAc)₂ and acridine as catalysts, yielding the compound as a colorless oil after purification . This compound is of interest in agrochemical and pharmaceutical research due to the electron-withdrawing chlorine substituent, which enhances reactivity in Michael addition or cycloaddition reactions .
Preparation Methods
Synthetic Routes and Reaction Conditions
Methyl (E)-3-(3-chlorophenyl)acrylate can be synthesized through the esterification of (E)-3-(3-chlorophenyl)acrylic acid with methanol in the presence of an acid catalyst such as sulfuric acid. The reaction typically occurs under reflux conditions to ensure complete conversion of the acid to the ester.
Industrial Production Methods
In an industrial setting, the production of this compound may involve continuous flow processes to enhance efficiency and yield. The use of automated systems for precise control of reaction parameters, such as temperature and pressure, can further optimize the production process.
Chemical Reactions Analysis
Types of Reactions
Methyl (E)-3-(3-chlorophenyl)acrylate undergoes various chemical reactions, including:
Oxidation: The compound can be oxidized to form corresponding carboxylic acids.
Reduction: Reduction reactions can convert the ester group to an alcohol.
Substitution: The chlorine atom on the phenyl ring can participate in nucleophilic substitution reactions.
Common Reagents and Conditions
Oxidation: Common oxidizing agents include potassium permanganate (KMnO4) and chromium trioxide (CrO3).
Reduction: Reducing agents such as lithium aluminum hydride (LiAlH4) or sodium borohydride (NaBH4) are typically used.
Substitution: Nucleophiles like sodium methoxide (NaOCH3) or potassium tert-butoxide (KOtBu) can be employed.
Major Products Formed
Oxidation: 3-(3-chlorophenyl)acrylic acid.
Reduction: Methyl (E)-3-(3-chlorophenyl)propanoate.
Substitution: Various substituted phenylacrylates depending on the nucleophile used.
Scientific Research Applications
Methyl (E)-3-(3-chlorophenyl)acrylate has several applications in scientific research:
Chemistry: It is used as an intermediate in the synthesis of more complex organic molecules.
Biology: The compound can be utilized in the study of enzyme-catalyzed reactions involving esters.
Industry: Used in the production of polymers and other materials with desirable properties.
Mechanism of Action
The mechanism by which methyl (E)-3-(3-chlorophenyl)acrylate exerts its effects depends on the specific reaction or application. In general, the ester group can undergo hydrolysis to release the corresponding acid and alcohol. The chlorine atom on the phenyl ring can influence the reactivity and selectivity of the compound in various chemical reactions.
Comparison with Similar Compounds
Comparison with Structural Analogues
Substituent Position and Electronic Effects
The position of the chlorine substituent significantly influences chemical behavior:
- Methyl (E)-3-(4-chlorophenyl)acrylate (para isomer): Exhibits distinct electronic properties due to the symmetrical para-chloro group. This isomer is used in crystallography studies and has been synthesized via similar Pd-catalyzed methods .
- Methyl (E)-3-(2-chlorophenyl)acrylate: The ortho-substituted derivative shows steric hindrance, affecting its reactivity in organometallic applications. Crystal structure analyses reveal altered dihedral angles compared to the meta isomer .
Functional Group Modifications
Trifluoromethyl Derivatives
- Methyl (E)-3-(3-chlorophenyl)-2-(trifluoromethyl)acrylate : The addition of a trifluoromethyl group at the α-position increases electrophilicity, making it suitable for radical polymerization or fluorinated material synthesis. This compound is synthesized via Pd(TFA)₂-mediated coupling in 1,4-dioxane, yielding E/Z isomer mixtures (e.g., 85% yield for 3e ).
- Methyl (E)-3-(3-methoxyphenyl)-2-(trifluoromethyl)acrylate : The methoxy group donates electrons, reducing reactivity compared to chloro-substituted analogues. Reported yields for such compounds reach 82% .
Cyano-Substituted Analogues
- (E)-Methyl 3-(3-chloro-4-methoxyphenyl)-2-cyanoacrylate: The cyano group enhances electrophilicity, favoring nucleophilic attacks. This derivative is used in high-throughput drug discovery due to its stability and versatility .
Steric and Physicochemical Properties
- Methyl (E)-3-(3,5-dichlorophenyl)acrylate : The dual chlorine substituents increase molecular weight (231.08 g/mol) and lipophilicity, improving membrane permeability in bioactive compounds. However, steric effects may reduce reaction yields .
- Methyl (2E)-3-(3-methylphenyl)acrylate : A methyl group at the meta position decreases polarity (MW 176.22 g/mol) and alters solubility profiles compared to chloro derivatives .
Biological Activity
Methyl (E)-3-(3-chlorophenyl)acrylate is an organic compound that has garnered attention for its potential biological activities, particularly in the fields of medicinal chemistry and material science. This article delves into the compound's biological activity, mechanisms of action, and relevant research findings, supported by data tables and case studies.
Chemical Structure and Properties
- IUPAC Name : this compound
- Molecular Formula : C10H9ClO2
- Structural Features : The compound features an acrylate functional group with a chlorophenyl substituent, characterized by a double bond in the E configuration, indicating that the higher priority substituents are on opposite sides of the double bond.
The biological activity of this compound is largely attributed to its ability to interact with various molecular targets, including enzymes and receptors. The presence of chlorine enhances its binding affinity, potentially increasing its specificity towards biological targets. The acrylate moiety allows for participation in chemical reactions that lead to the formation of active intermediates with biological effects.
1. Antiviral Activity
Recent studies have demonstrated that derivatives of this compound exhibit antiviral properties. For example, in vitro assays revealed that certain substituted analogs could inhibit the replication of viruses such as Chikungunya virus (CHIKV). The antiviral activity was assessed at a concentration of 20 µM, showing varying degrees of efficacy depending on the specific substitutions on the phenyl ring .
2. Cytotoxicity Studies
Cytotoxicity assays performed on Vero E6 cells indicated that some derivatives were highly cytotoxic, with cell viability dropping below 50% at certain concentrations. For instance, one derivative exhibited a cell viability of only 41.5% after 48 hours at a concentration of 20 µM. In contrast, other derivatives maintained higher cell viability while still demonstrating antiviral activity .
Data Table: Biological Activity Summary
Compound | Activity Type | Concentration (µM) | Cell Viability (%) | Inhibition (%) |
---|---|---|---|---|
LQM328 | Antiviral | 20 | 75.5 | 33.1 |
LQM331 | Antiviral | 20 | >90 | 0 |
LQM334 | Antiviral | 40 | >95 | 81.1 |
LQM329 | Cytotoxic | 20 | 41.5 | - |
Case Study 1: Antiviral Evaluation
In a study evaluating the antiviral efficacy of various acrylamide derivatives related to this compound, researchers found that modifying the position of chlorine atoms significantly affected both cytotoxicity and viral inhibition rates. For instance, shifting chlorine from position 3 to position 4 resulted in a non-cytotoxic compound that was inactive against CHIKV, while retaining it at position 3 led to improved antiviral properties .
Case Study 2: Structure-Activity Relationship (SAR)
A detailed structure-activity relationship analysis highlighted how different substituents on the phenyl ring influenced both cytotoxicity and antiviral activity. Compounds with electron-withdrawing groups showed enhanced biological activity compared to those with electron-donating groups. This insight is crucial for designing more effective therapeutic agents based on this compound .
Q & A
Basic Research Questions
Q. What are the common synthetic routes for Methyl (E)-3-(3-chlorophenyl)acrylate, and how can reaction conditions be optimized for higher yields?
- Methodological Answer : A widely used approach involves esterification of (E)-3-(3-chlorophenyl)acrylic acid with methanol under acidic catalysis. For example, a 73% yield was achieved by reacting the acrylic acid derivative with methanol in the presence of a Brønsted acid catalyst (e.g., H₂SO₄) under reflux conditions . Key optimization parameters include:
- Catalyst concentration : Higher acid concentrations accelerate esterification but may promote side reactions.
- Temperature : Controlled reflux (e.g., 60–80°C) balances reaction rate and thermal stability.
- Solvent choice : Polar aprotic solvents (e.g., DMF) improve solubility of aromatic intermediates.
Parallel methods include Pd-catalyzed cross-couplings (e.g., Heck reactions) for introducing the 3-chlorophenyl moiety .
Q. Which spectroscopic techniques are most effective for confirming the structure of this compound?
- Methodological Answer :
- ¹H/¹³C NMR :
- ¹H NMR : Expect signals at δ 6.3–6.5 ppm (trans-vinyl protons, J ≈ 16 Hz) and δ 3.7–3.8 ppm (ester methyl group). Aromatic protons (3-chlorophenyl) appear as multiplets in δ 7.2–7.5 ppm .
- ¹³C NMR : The ester carbonyl resonates at δ 165–170 ppm, with vinyl carbons at δ 120–140 ppm .
- IR Spectroscopy : Strong C=O stretch (~1700 cm⁻¹) and conjugated C=C stretch (~1630 cm⁻¹) confirm the acrylate backbone .
- HRMS : Molecular ion peaks (e.g., [M+H]⁺) should match the theoretical mass (C₁₀H₉ClO₂, 196.03 g/mol) within 5 ppm error .
Q. What are the critical parameters for achieving high purity in the synthesis of this compound?
- Methodological Answer :
- Recrystallization : Use a solvent pair like ethanol/water to remove unreacted starting materials. Cooling rates ≤2°C/min minimize impurity trapping .
- Column Chromatography : Employ silica gel with hexane/ethyl acetate (4:1) for optimal separation of ester products from chlorinated byproducts.
- Melting Point Analysis : Pure samples exhibit sharp melting points (e.g., 278.3–278.9°C for the 3-chlorophenyl derivative). Deviations >2°C suggest impurities .
Advanced Research Questions
Q. How can single-crystal X-ray diffraction (SCXRD) resolve ambiguities in the stereochemistry of this compound?
- Methodological Answer : SCXRD with programs like SHELXL determines the E-configuration by measuring dihedral angles between the acrylate and aryl groups. For example:
- Key Metrics :
- Torsion angle between C1-C2-C3-C4 should approach 180° (trans configuration).
- Packing analysis reveals intermolecular interactions (e.g., π-π stacking) stabilizing the E-form .
- Practical Workflow :
Grow crystals via slow evaporation in dichloromethane/hexane.
Collect diffraction data (Mo-Kα radiation, λ = 0.71073 Å).
Refine using SHELXL with R-factor <0.05 for high confidence .
Q. How to address discrepancies in melting points across different synthesis batches?
- Methodological Answer :
- Purity Assessment :
- HPLC : Use a C18 column (acetonitrile/water gradient) to quantify impurities.
- DSC : Detect polymorphic transitions (e.g., endothermic peaks) that alter melting behavior.
- Crystallization Conditions :
- Slow cooling rates yield thermodynamically stable polymorphs.
- Solvent polarity adjustments (e.g., switching from ethanol to acetone) can suppress impurity incorporation .
Q. What catalytic systems enhance the stereoselective synthesis of this compound?
- Methodological Answer :
- Pd-Catalyzed Heck Reactions :
- Use Pd(OAc)₂ with PPh₃ ligands in DMF at 80°C to couple 3-chlorophenylboronic acid with methyl acrylate.
- Achieve >90% E-selectivity via bulky ligands (e.g., t-Bu₃P) that sterically hinder the Z-pathway .
- Organocatalysis :
- Proline-derived catalysts promote asymmetric Michael additions to α,β-unsaturated esters, though yields are lower (~50%) .
Q. How can computational methods predict the reactivity of this compound in nucleophilic additions?
- Methodological Answer :
- DFT Calculations :
Optimize geometry at the B3LYP/6-31G(d) level.
Calculate Fukui indices to identify electrophilic sites (β-carbon of acrylate is most reactive).
Properties
IUPAC Name |
methyl 3-(3-chlorophenyl)prop-2-enoate | |
---|---|---|
Details | Computed by LexiChem 2.6.6 (PubChem release 2019.06.18) | |
Source | PubChem | |
URL | https://pubchem.ncbi.nlm.nih.gov | |
Description | Data deposited in or computed by PubChem | |
InChI |
InChI=1S/C10H9ClO2/c1-13-10(12)6-5-8-3-2-4-9(11)7-8/h2-7H,1H3 | |
Details | Computed by InChI 1.0.5 (PubChem release 2019.06.18) | |
Source | PubChem | |
URL | https://pubchem.ncbi.nlm.nih.gov | |
Description | Data deposited in or computed by PubChem | |
InChI Key |
QCCTYRQOMYMCCU-UHFFFAOYSA-N | |
Details | Computed by InChI 1.0.5 (PubChem release 2019.06.18) | |
Source | PubChem | |
URL | https://pubchem.ncbi.nlm.nih.gov | |
Description | Data deposited in or computed by PubChem | |
Canonical SMILES |
COC(=O)C=CC1=CC(=CC=C1)Cl | |
Details | Computed by OEChem 2.1.5 (PubChem release 2019.06.18) | |
Source | PubChem | |
URL | https://pubchem.ncbi.nlm.nih.gov | |
Description | Data deposited in or computed by PubChem | |
Molecular Formula |
C10H9ClO2 | |
Details | Computed by PubChem 2.1 (PubChem release 2019.06.18) | |
Source | PubChem | |
URL | https://pubchem.ncbi.nlm.nih.gov | |
Description | Data deposited in or computed by PubChem | |
Molecular Weight |
196.63 g/mol | |
Details | Computed by PubChem 2.1 (PubChem release 2021.05.07) | |
Source | PubChem | |
URL | https://pubchem.ncbi.nlm.nih.gov | |
Description | Data deposited in or computed by PubChem | |
Disclaimer and Information on In-Vitro Research Products
Please be aware that all articles and product information presented on BenchChem are intended solely for informational purposes. The products available for purchase on BenchChem are specifically designed for in-vitro studies, which are conducted outside of living organisms. In-vitro studies, derived from the Latin term "in glass," involve experiments performed in controlled laboratory settings using cells or tissues. It is important to note that these products are not categorized as medicines or drugs, and they have not received approval from the FDA for the prevention, treatment, or cure of any medical condition, ailment, or disease. We must emphasize that any form of bodily introduction of these products into humans or animals is strictly prohibited by law. It is essential to adhere to these guidelines to ensure compliance with legal and ethical standards in research and experimentation.