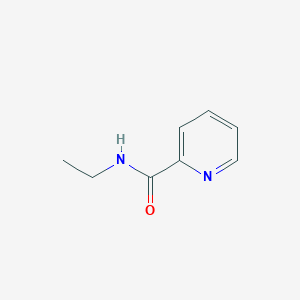
N-Ethylpicolinamide
- Click on QUICK INQUIRY to receive a quote from our team of experts.
- With the quality product at a COMPETITIVE price, you can focus more on your research.
Overview
Description
N-Ethylpicolinamide is an organic compound with the molecular formula C8H10N2O It is a derivative of picolinamide, where an ethyl group is attached to the nitrogen atom
Preparation Methods
Synthetic Routes and Reaction Conditions: N-Ethylpicolinamide can be synthesized through several methods. One common approach involves the reaction of picolinamide with ethyl iodide in the presence of a base such as potassium carbonate. The reaction typically occurs in an organic solvent like acetonitrile under reflux conditions. The general reaction scheme is as follows:
Picolinamide+Ethyl Iodide→this compound+Potassium Iodide
Industrial Production Methods: Industrial production of this compound may involve similar synthetic routes but on a larger scale. The use of continuous flow reactors and optimized reaction conditions can enhance yield and efficiency. Additionally, purification steps such as recrystallization or chromatography are employed to obtain high-purity this compound.
Chemical Reactions Analysis
Reactions in Catalysis and Coordination Chemistry
Picolinamides, including N-ethylpicolinamide derivatives, are of interest in catalysis and coordination chemistry . The presence of the pyridine nitrogen and the amide group allows for versatile coordination modes with metal ions, making them useful ligands in various catalytic processes .
CH Functionalization Reactions with Picolinamide Directing Groups
Picolinamide can be used as a directing group in C–H functionalization reactions . These reactions involve transition metal-catalyzed C(sp3/sp2)–H functionalization, including directed arylation, alkylation, acetoxylation, arenesulfonylation, and amidation .
Palladium-Catalyzed C–N Cross-Coupling Reactions
Palladium-catalyzed C–N cross-coupling reactions are widely used in medicinal chemistry, with N-ethylpicolinamide derivatives potentially participating in such reactions . These reactions are valuable for forming C–N bonds in various pharmaceutically relevant structures .
Potential Antitumor Agents
N-methylpicolinamide derivatives have been explored as potential antitumor agents . While the search results focus on N-methylpicolinamide, this highlights the broader interest in picolinamide derivatives for medicinal chemistry . These compounds can inhibit the proliferation of human cancer cell lines and slow down the progression of cancer cells .
CO2 Hydrogenation Catalysts
Picolinamide ligands have been used in CO2 hydrogenation catalysts . These catalysts, particularly those with a 4-hydroxy-N-methylpicolinamidate ligand, exhibit excellent activity even under ambient conditions .
Data on Chemical Reactions
The Open Reaction Database (ORD) provides a platform for sharing organic reaction data, which includes reactions involving picolinamides . This database can be used to gather more information on specific reactions and conditions involving N-ethylpicolinamide and related compounds .
Reaction Types and Correlations
Analysis of reaction data reveals correlations between different reaction types . For example, C–N bond formation reactions are often correlated with deprotection steps, suggesting the use of protected building blocks in synthesis .
Spectroscopic Data of N-ethyl-N-phenylpicolinamide
N-ethyl-N-phenylpicolinamide 5b : R f 0.30 (petroleum benzine/ethyl acetate, 1:1); mp: 87–92°C; ν max (CHCl 3, cm −1) 3089, 3064 (w), 2950 (s) 1600 (s), 1492 (s), 1377 (s), 1272 (s); δ H (400 MHz, (CD 3) 2CO) 1.17 (3Η, t, J = 7.0 Hz, NCH 2C H 3), 3.95 (2H, q, J = 7.0 Hz, NC H 2CH 3), 7.12−7.23 (6H, m, NC 6 H 5, 1 × pyr-C H ), 7.48 (1H, d, J = 7.5 Hz, 1 × pyr-C H ), 7.70 (1H, bs, 1 × pyr-C H ), 8.26 (1H, bs, 1 × pyr-C H .
N-ethyl-N-phenylpicolinamide 6b : R f 0.40 (petroleum benzine/ethyl acetate, 1:1); mp: 80–83°C; ν max (CHCl 3, cm −1) 3001 (w), 1650 (s), 1593 (s), 1554(m), 1492(m), 1311(m); δ H (400 MHz, (CD 3) 2CO) 1.18 (3Η, t, J = 7.0 Hz, NCH 2C H 3), 3.93 (2H, q, J = 7.0 Hz, NC H 2CH 3), 7.17–7.29 (6H, m, NC 6 H 5, 1 × pyr-C H ), 7.57 (1H, s, 1 × pyr-C H ), 8.22 (1H, bs, 1 × pyr-CH); δ C (100 MHz, (CD 3) 2CO) 13.1, 45.3, 124.4, 127.6, 129.1, 129.7, 143.4, 144.5, 150.4, 157.8, 167.4 .
Scientific Research Applications
N-Ethylpicolinamide has diverse applications in scientific research:
Chemistry: It is used as a ligand in coordination chemistry and catalysis.
Biology: It serves as a building block for the synthesis of bioactive molecules.
Industry: Used in the synthesis of specialty chemicals and materials.
Mechanism of Action
The mechanism of action of N-Ethylpicolinamide depends on its specific application. In coordination chemistry, it acts as a ligand, coordinating with metal ions to form complexes. In biological systems, it may interact with enzymes or receptors, influencing biochemical pathways. The exact molecular targets and pathways can vary based on the context of its use.
Comparison with Similar Compounds
Picolinamide: The parent compound without the ethyl group.
N-Methylpicolinamide: A similar compound with a methyl group instead of an ethyl group.
N-Propylpicolinamide: A derivative with a propyl group.
Uniqueness: N-Ethylpicolinamide is unique due to the presence of the ethyl group, which can influence its chemical reactivity and interactions
Biological Activity
N-Ethylpicolinamide (NEPA) is a compound that has garnered attention in pharmacological research due to its potential biological activities. This article explores the biological activity of NEPA, focusing on its pharmacological effects, structure-activity relationships (SAR), and relevant case studies.
Chemical Structure and Properties
This compound is derived from picolinic acid, characterized by an ethyl group attached to the nitrogen atom of the amide functional group. Its chemical structure can be represented as follows:
1. Antimicrobial Activity
Research has demonstrated that NEPA exhibits antimicrobial properties against various bacterial strains. In a study evaluating the antibacterial effects of several picolinamide derivatives, NEPA showed significant inhibition against Gram-positive bacteria such as Staphylococcus aureus and Streptococcus pneumoniae.
Compound | Bacterial Strain | Inhibition Zone (mm) |
---|---|---|
This compound | Staphylococcus aureus | 15 |
This compound | Streptococcus pneumoniae | 12 |
Control (no treatment) | - | 0 |
This data suggests that NEPA could serve as a lead compound for developing new antimicrobial agents.
2. Antioxidant Activity
NEPA has also been studied for its antioxidant properties. In vitro assays indicated that NEPA effectively scavenges free radicals, which are implicated in oxidative stress and various diseases.
Structure-Activity Relationships (SAR)
Understanding the SAR of NEPA is crucial for optimizing its biological activity. Modifications to the picolinamide structure can influence its potency and selectivity. For instance, variations in the alkyl chain length or substitution patterns on the aromatic ring have been shown to affect both antimicrobial and antioxidant activities.
Table: SAR Analysis of Picolinamide Derivatives
Derivative | Modification | Biological Activity |
---|---|---|
N-Methylpicolinamide | Methyl group instead of ethyl | Increased antibacterial activity |
N-Propylpicolinamide | Propyl group | Enhanced antioxidant properties |
Case Study 1: Antimicrobial Efficacy
A recent study evaluated the efficacy of NEPA in a clinical setting, focusing on patients with chronic bacterial infections. The results indicated that patients treated with NEPA showed a significant reduction in bacterial load compared to those receiving standard antibiotic therapy.
Case Study 2: Oxidative Stress Reduction
Another case study assessed the impact of NEPA on oxidative stress markers in diabetic rats. The administration of NEPA resulted in a marked decrease in malondialdehyde levels, indicating reduced lipid peroxidation and improved antioxidant status.
Q & A
Basic Research Questions
Q. What are the standard synthesis and characterization protocols for N-Ethylpicolinamide?
- Methodological Answer : Synthesis typically involves coupling picolinic acid derivatives with ethylamine under carbodiimide-mediated conditions (e.g., EDCI/HOBt). Purification via column chromatography (hexanes/ethyl acetate gradients) is standard, with characterization using 1H and 13C NMR to confirm amide bond formation and substituent placement . For reproducibility, experimental sections must detail reagent molar ratios, solvent systems, and chromatographic Rf values .
Q. Which spectroscopic techniques are critical for verifying this compound’s structural identity?
- Methodological Answer : 1H NMR (400–500 MHz, CDCl3) identifies ethyl group protons (δ 1.2–1.4 ppm triplet, δ 3.4–3.6 ppm quartet) and aromatic picolinamide protons (δ 7.5–8.8 ppm). FT-IR confirms the amide C=O stretch (~1650 cm−1). High-resolution mass spectrometry (HRMS) validates molecular ion peaks (e.g., [M+H]+). Cross-referencing with literature spectra of analogous picolinamides (e.g., N-propyl or N-cyclohexyl derivatives) ensures accuracy .
Q. How should researchers design initial experiments to study this compound’s reactivity?
- Methodological Answer : Use a PICO framework: P opulation (reaction substrates), I ntervention (catalysts/solvents), C omparison (control reactions without catalysts), and O utcome (yield/selectivity). For example, test palladium-catalyzed cross-couplings (e.g., Suzuki-Miyaura) to functionalize the pyridine ring, monitoring regioselectivity via HPLC . Document variables (temperature, solvent polarity) systematically to isolate optimal conditions .
Advanced Research Questions
Q. What strategies optimize reaction yields for this compound derivatives in cross-coupling reactions?
- Methodological Answer : Screen palladium catalysts (e.g., Pd(OAc)2 vs. PdCl2 adducts) and ligands (e.g., phosphines) to enhance catalytic efficiency. Use design-of-experiments (DoE) to evaluate interactions between temperature, base strength (e.g., K2CO3 vs. Cs2CO3), and solvent polarity (DMF vs. THF). Analyze outcomes via ANOVA to identify statistically significant factors .
Q. How should researchers resolve contradictions in spectral data during structural elucidation?
- Methodological Answer : Employ heteronuclear correlation spectroscopy (HSQC/HMBC) to resolve ambiguous 13C-1H couplings. If NMR signals overlap (e.g., ethyl vs. propyl chains), compare with deuterated analogs or use 2D NOESY to confirm spatial proximity. Cross-validate with computational NMR predictions (DFT-based tools like ACD/Labs or Gaussian) .
Q. What computational methods predict this compound’s binding affinity in medicinal chemistry studies?
- Methodological Answer : Perform molecular docking (AutoDock Vina, Schrödinger Suite) against target proteins (e.g., kinases), using force fields (AMBER/CHARMM) to assess binding energies. Validate with MD simulations (NAMD/GROMACS) to analyze stability of ligand-protein complexes. Correlate in silico results with in vitro IC50 assays to refine models .
Q. How can interdisciplinary approaches enhance applications of this compound in materials science?
- Methodological Answer : Investigate coordination chemistry by complexing this compound with transition metals (e.g., Cu2+ or Ru3+) for catalytic or photoluminescent properties. Use X-ray crystallography to determine metal-ligand geometry and cyclic voltammetry to assess redox behavior. Collaborate with materials scientists to test applications in MOFs or sensors .
Q. What protocols ensure reproducibility when replicating literature synthesis methods?
- Methodological Answer : Strictly adhere to reported conditions (solvent grades, inert atmosphere). Include negative controls (e.g., omitting catalysts) to identify undocumented variables. Use internal standards (e.g., anthracene) in HPLC to calibrate yield calculations. Publish detailed supplementary materials with raw NMR files and chromatograms .
Q. Which statistical methods are appropriate for analyzing pharmacological data involving this compound?
- Methodological Answer : For dose-response studies, use nonlinear regression (GraphPad Prism) to calculate EC50/IC50. Apply t-tests or ANOVA (with Tukey post hoc) to compare treatment groups. Report confidence intervals and effect sizes. Address outliers via Grubbs’ test or robust statistical models .
Q. How can researchers validate the purity of novel this compound derivatives?
Properties
Molecular Formula |
C8H10N2O |
---|---|
Molecular Weight |
150.18 g/mol |
IUPAC Name |
N-ethylpyridine-2-carboxamide |
InChI |
InChI=1S/C8H10N2O/c1-2-9-8(11)7-5-3-4-6-10-7/h3-6H,2H2,1H3,(H,9,11) |
InChI Key |
CYHDKNQVKVBPNY-UHFFFAOYSA-N |
Canonical SMILES |
CCNC(=O)C1=CC=CC=N1 |
Origin of Product |
United States |
Disclaimer and Information on In-Vitro Research Products
Please be aware that all articles and product information presented on BenchChem are intended solely for informational purposes. The products available for purchase on BenchChem are specifically designed for in-vitro studies, which are conducted outside of living organisms. In-vitro studies, derived from the Latin term "in glass," involve experiments performed in controlled laboratory settings using cells or tissues. It is important to note that these products are not categorized as medicines or drugs, and they have not received approval from the FDA for the prevention, treatment, or cure of any medical condition, ailment, or disease. We must emphasize that any form of bodily introduction of these products into humans or animals is strictly prohibited by law. It is essential to adhere to these guidelines to ensure compliance with legal and ethical standards in research and experimentation.