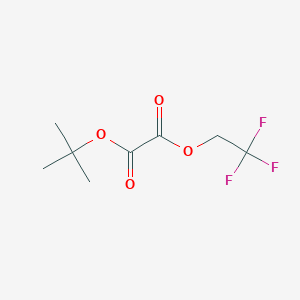
Tert-butyl 2,2,2-trifluoroethyl oxalate
Overview
Description
Tert-butyl 2,2,2-trifluoroethyl oxalate: is an organic compound with the molecular formula C8H11F3O4. It is a liquid at room temperature and is known for its unique chemical properties due to the presence of both tert-butyl and trifluoroethyl groups. This compound is used in various chemical reactions and has applications in scientific research and industrial processes.
Preparation Methods
Synthetic Routes and Reaction Conditions: The synthesis of tert-butyl 2,2,2-trifluoroethyl oxalate typically involves the esterification of oxalic acid with tert-butyl alcohol and 2,2,2-trifluoroethanol. The reaction is usually carried out in the presence of a strong acid catalyst such as sulfuric acid or p-toluenesulfonic acid. The reaction conditions include maintaining a temperature range of 60-80°C and continuous removal of water to drive the reaction to completion.
Industrial Production Methods: On an industrial scale, the production of this compound follows similar principles but may involve more efficient catalysts and optimized reaction conditions to increase yield and purity. The use of continuous flow reactors and advanced separation techniques such as distillation and crystallization are common in industrial settings.
Chemical Reactions Analysis
Types of Reactions: Tert-butyl 2,2,2-trifluoroethyl oxalate undergoes various chemical reactions, including:
Hydrolysis: In the presence of water and an acid or base catalyst, it can hydrolyze to form oxalic acid, tert-butyl alcohol, and 2,2,2-trifluoroethanol.
Reduction: It can be reduced using reducing agents like lithium aluminum hydride to form the corresponding alcohols.
Substitution: The trifluoroethyl group can undergo nucleophilic substitution reactions with nucleophiles such as amines and thiols.
Common Reagents and Conditions:
Hydrolysis: Acidic or basic conditions, water, temperature range of 50-70°C.
Reduction: Lithium aluminum hydride, dry ether, temperature range of 0-25°C.
Substitution: Nucleophiles (amines, thiols), solvents like dichloromethane, temperature range of 20-40°C.
Major Products:
Hydrolysis: Oxalic acid, tert-butyl alcohol, 2,2,2-trifluoroethanol.
Reduction: Corresponding alcohols.
Substitution: Substituted products with nucleophiles.
Scientific Research Applications
Tert-butyl 2,2,2-trifluoroethyl oxalate has several applications in scientific research:
Biology: Employed in the study of enzyme-catalyzed reactions involving ester hydrolysis.
Medicine: Investigated for its potential use in drug development due to its unique chemical properties.
Industry: Utilized in the production of specialty chemicals and materials with specific properties.
Mechanism of Action
The mechanism of action of tert-butyl 2,2,2-trifluoroethyl oxalate involves its ability to undergo ester hydrolysis and other chemical reactions. The molecular targets include enzymes that catalyze ester hydrolysis, leading to the formation of oxalic acid, tert-butyl alcohol, and 2,2,2-trifluoroethanol. The pathways involved in these reactions are typically those associated with ester hydrolysis and nucleophilic substitution.
Comparison with Similar Compounds
Bis(2,2,2-trifluoroethyl) oxalate: Similar structure but with two trifluoroethyl groups instead of one tert-butyl and one trifluoroethyl group.
Tert-butyl oxalate: Contains two tert-butyl groups instead of one tert-butyl and one trifluoroethyl group.
Uniqueness: Tert-butyl 2,2,2-trifluoroethyl oxalate is unique due to the presence of both tert-butyl and trifluoroethyl groups, which impart distinct chemical properties. The trifluoroethyl group enhances the compound’s reactivity and stability, while the tert-butyl group provides steric hindrance, making it useful in selective reactions.
Properties
IUPAC Name |
2-O-tert-butyl 1-O-(2,2,2-trifluoroethyl) oxalate | |
---|---|---|
Source | PubChem | |
URL | https://pubchem.ncbi.nlm.nih.gov | |
Description | Data deposited in or computed by PubChem | |
InChI |
InChI=1S/C8H11F3O4/c1-7(2,3)15-6(13)5(12)14-4-8(9,10)11/h4H2,1-3H3 | |
Source | PubChem | |
URL | https://pubchem.ncbi.nlm.nih.gov | |
Description | Data deposited in or computed by PubChem | |
InChI Key |
VAYBILISVGIYDM-UHFFFAOYSA-N | |
Source | PubChem | |
URL | https://pubchem.ncbi.nlm.nih.gov | |
Description | Data deposited in or computed by PubChem | |
Canonical SMILES |
CC(C)(C)OC(=O)C(=O)OCC(F)(F)F | |
Source | PubChem | |
URL | https://pubchem.ncbi.nlm.nih.gov | |
Description | Data deposited in or computed by PubChem | |
Molecular Formula |
C8H11F3O4 | |
Source | PubChem | |
URL | https://pubchem.ncbi.nlm.nih.gov | |
Description | Data deposited in or computed by PubChem | |
Molecular Weight |
228.17 g/mol | |
Source | PubChem | |
URL | https://pubchem.ncbi.nlm.nih.gov | |
Description | Data deposited in or computed by PubChem | |
Retrosynthesis Analysis
AI-Powered Synthesis Planning: Our tool employs the Template_relevance Pistachio, Template_relevance Bkms_metabolic, Template_relevance Pistachio_ringbreaker, Template_relevance Reaxys, Template_relevance Reaxys_biocatalysis model, leveraging a vast database of chemical reactions to predict feasible synthetic routes.
One-Step Synthesis Focus: Specifically designed for one-step synthesis, it provides concise and direct routes for your target compounds, streamlining the synthesis process.
Accurate Predictions: Utilizing the extensive PISTACHIO, BKMS_METABOLIC, PISTACHIO_RINGBREAKER, REAXYS, REAXYS_BIOCATALYSIS database, our tool offers high-accuracy predictions, reflecting the latest in chemical research and data.
Strategy Settings
Precursor scoring | Relevance Heuristic |
---|---|
Min. plausibility | 0.01 |
Model | Template_relevance |
Template Set | Pistachio/Bkms_metabolic/Pistachio_ringbreaker/Reaxys/Reaxys_biocatalysis |
Top-N result to add to graph | 6 |
Feasible Synthetic Routes
Disclaimer and Information on In-Vitro Research Products
Please be aware that all articles and product information presented on BenchChem are intended solely for informational purposes. The products available for purchase on BenchChem are specifically designed for in-vitro studies, which are conducted outside of living organisms. In-vitro studies, derived from the Latin term "in glass," involve experiments performed in controlled laboratory settings using cells or tissues. It is important to note that these products are not categorized as medicines or drugs, and they have not received approval from the FDA for the prevention, treatment, or cure of any medical condition, ailment, or disease. We must emphasize that any form of bodily introduction of these products into humans or animals is strictly prohibited by law. It is essential to adhere to these guidelines to ensure compliance with legal and ethical standards in research and experimentation.