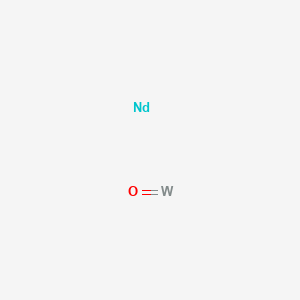
Neodymium tungsten oxide
- Click on QUICK INQUIRY to receive a quote from our team of experts.
- With the quality product at a COMPETITIVE price, you can focus more on your research.
Overview
Description
Neodymium tungsten oxide (Nd-doped tungsten oxide or Nd-W-O systems) is a specialized material combining the unique properties of neodymium oxide (Nd₂O₃) and tungsten oxides (WO₃₋ₓ). Neodymium oxide, a rare earth compound, is characterized by its high melting point (2233°C), thermal stability, and optical properties . When integrated into tungsten oxide matrices, Nd₂O₃ enhances structural durability, radiation shielding, and catalytic performance . Applications span advanced plasma-facing materials, solid-state lasers, and gamma radiation shielding glasses .
Preparation Methods
Neodymium tungsten oxide can be synthesized through different methods:
Reaction of Neodymium (III) Oxide and Tungsten (VI) Oxide: This method involves reacting neodymium (III) oxide (Nd₂O₃) with tungsten (VI) oxide (WO₃) at a temperature of 1000°C to produce anhydrous this compound. [ \text{Nd}_2\text{O}_3 + 3 \text{WO}_3 \rightarrow \text{Nd}_2(\text{WO}_4)_3 ]
Reaction of Sodium Tungstate and Neodymium (III) Nitrate: The nonahydrate form of this compound can be produced by reacting sodium tungstate (Na₂WO₄) with neodymium (III) nitrate (Nd(NO₃)₃) in an aqueous solution. [ 3 \text{Na}_2\text{WO}_4 + 2 \text{Nd}(\text{NO}_3)_3 \rightarrow \text{Nd}_2(\text{WO}_4)_3 \cdot 9\text{H}_2\text{O} + 6 \text{NaNO}_3 ]
Chemical Reactions Analysis
Neodymium tungsten oxide undergoes various chemical reactions, including:
Oxidation and Reduction: The compound can participate in redox reactions, where it can either gain or lose electrons.
Substitution Reactions: this compound can undergo substitution reactions where one or more of its atoms are replaced by other atoms or groups.
Hydration and Dehydration: The compound can form hydrates, such as the nonahydrate form, and can lose water molecules upon heating.
Common reagents used in these reactions include sodium tungstate, neodymium (III) nitrate, and tungsten (VI) oxide. The major products formed from these reactions include anhydrous and hydrated forms of this compound.
Scientific Research Applications
Neodymium tungsten oxide is a compound with various applications, particularly in areas involving rare earth element utilization and advanced material science. It is often explored for its unique optical and electrical properties, which are beneficial in specialized technological applications .
Scientific Research Applications
Material Science and Engineering
- Electroplating: Neodymium-nickel-tungsten electroplating solutions benefit from the inclusion of neodymium oxide. These solutions are designed to minimize environmental damage, provide long-term storage stability post-preparation, and offer high current efficiency. The resulting coatings are non-microcracked and exhibit good corrosion resistance, making them suitable for surface treatment in petroleum machinery, where anti-corrosion and anti-wear properties are crucial .
- Tungsten Bronze Structures: this compound can be incorporated into tungsten bronze-type compounds (M<sub>x</sub>WO<sub>3</sub>, where M = Cs, Rb, K, Na, and NH<sub>4</sub>). Doping with elements like neodymium enhances broadband near-infrared (NIR) absorption properties, which are valuable in various applications .
Industrial Applications
- Glass Production: Neodymium oxide (Nd<sub>2</sub>O<sub>3</sub>), a related compound, is used in glass coloring and for decolorizing ferrous glass. It serves as an additive for UV-absorbing glass (solar control glass) and is a component in neodymium YAG lasers, which are widely used industrially . Neodymium salts are also used to color enamels and in mixtures for ceramic pigments .
- DC Arc Cathodes: Neodymium oxide-containing tungsten is used as a cathode for DC arcs in argon atmospheres. Studies have shown that using neodymium oxide-containing tungsten results in a lower erosion rate compared to conventional thorium oxide-containing tungsten. The reduced erosion rate is attributed to the lower surface temperature of the cathode due to the lower melting point of neodymium oxide .
Photothermal Materials
- NIR Absorption: Tungsten-oxide-based materials, including those modified with neodymium, have garnered attention for their ability to absorb near-infrared (NIR) light and efficiently convert it into heat . This is due to their oxygen defect structure and strong local surface plasma resonance (LSPR), which provides strong photoabsorption across a broad NIR wavelength range .
- Smart Windows and Electrochromic Devices: Oxygen-deficient WO<sub>2.72</sub>, a related tungsten oxide, is used in smart windows, electrochromic devices, photothermal therapy, and NIR shielding . The integration of neodymium can potentially enhance these properties .
Mechanism of Action
The mechanism by which neodymium tungsten oxide exerts its effects involves its ability to absorb and convert light energy into heat. This property is particularly useful in photothermal therapy, where the compound can generate localized heat to destroy cancer cells. The molecular targets and pathways involved include the absorption of near-infrared light and the generation of reactive oxygen species that can damage cellular components .
Comparison with Similar Compounds
Structural and Chemical Properties
Neodymium Oxide (Nd₂O₃) vs. Cerium Oxide (CeO₂)
- Structure : Both Nd₂O₃ and CeO₂ adopt cubic fluorite structures but differ in lattice parameters due to ionic radius variations (Nd³⁺: 0.98 Å; Ce⁴⁺: 0.87 Å).
- Reactivity : Nd₂O₃ exhibits slower carbochlorination kinetics compared to CeO₂, with pore diffusion being the rate-limiting step for Nd₂O₃, while CeO₂ reactions are surface-controlled .
- Thermal Stability : Nd₂O₃ has a higher melting point (2233°C) than CeO₂ (2400°C), but CeO₂ shows superior oxygen storage capacity due to redox-active Ce⁴⁺/Ce³⁺ transitions .
Tungsten Oxides (WO₃₋ₓ) vs. Neodymium Tungsten Oxide
- Non-Stoichiometry: Pure tungsten oxides (e.g., WO₃, W₁₈O₄₉) exhibit oxygen vacancies (Ovacs) that enhance electrical conductivity and electrochromic properties. Nd doping reduces Ovac density, improving thermal stability but reducing conductivity .
- Synthesis : Tungsten oxides are often synthesized via solvothermal or plasma-based methods , whereas Nd-W-O systems require co-precipitation or spark plasma sintering (SPS) to ensure molecular-level homogeneity .
Functional Performance
Radiation Shielding
- Nd₂O₃-doped glasses (e.g., VTBNd1.5) outperform boron (III) oxide in gamma attenuation due to Nd's high electron density (1.5 mol% Nd₂O₃ achieves optimal shielding) .
- Comparatively, tungsten oxides like WO₃ are used in neutron shielding but lack rare earth elements' photon absorption efficiency .
Mechanical and Thermal Properties
- ODS Tungsten Alloys : Nd₂O₃ and CeO₂ are both used in oxide dispersion-strengthened (ODS) tungsten. Nd₂O₃ provides finer grain structures (0.5–1.2 µm) and higher Vickers hardness (520 HV) compared to CeO₂-doped samples (480 HV) .
- Thermal Conductivity : Nd₂O₃-ODS tungsten exhibits 15% higher thermal conductivity (173 W/m·K) than CeO₂-ODS variants, critical for plasma-facing materials .
Toxicity and Environmental Impact
- Nd₂O₃ : Inhalation risks include lung injury and fibrosis, necessitating controlled handling .
- WO₃: Generally low toxicity but poses environmental risks during nanoparticle synthesis .
- Recycling : Nd₂O₃ recovery from magnets via electrolysis reduces environmental impact by 90% compared to primary production, whereas tungsten recycling remains energy-intensive .
Data Tables
Table 1: Thermal and Mechanical Properties of Selected Oxides
Property | Nd₂O₃ | CeO₂ | WO₃ |
---|---|---|---|
Melting Point (°C) | 2233 | 2400 | 1473 |
Thermal Conductivity (W/m·K) | 13.5 | 10.2 | 20–30 |
Vickers Hardness (HV) | 520 (ODS-W) | 480 (ODS-W) | N/A |
Carbochlorination Rate | Pore-limited | Surface-limited | N/A |
Biological Activity
Neodymium tungsten oxide (NdWO₄) is a compound that has garnered attention due to its potential applications in various fields, including electronics and catalysis. However, its biological activity remains under-researched. This article aims to synthesize existing knowledge on the biological implications of this compound, focusing on its toxicity, antibacterial properties, and potential therapeutic applications.
This compound is a rare earth metal oxide that combines neodymium (Nd) and tungsten (W) in a specific stoichiometric ratio. Its unique properties stem from both the neodymium and tungsten components, which can influence its behavior in biological systems.
General Toxicity
Research indicates that compounds containing tungsten, including tungsten oxide, exhibit varying degrees of toxicity depending on their form and exposure routes. For instance, studies have shown that tungsten oxide nanoparticles can induce pulmonary toxicity and genotoxic effects upon inhalation. A study reported a no-observed-adverse-effect concentration (NOAEC) of 5 mg/m³ for tungsten oxide nanoparticles in hamsters, while a lower observable adverse effect concentration (LOAEC) was found at 63 mg/m³ in rats .
Neodymium Toxicity
Neodymium compounds also pose health risks. The toxicity profile of neodymium suggests that it can lead to adverse effects in high concentrations, although specific data on this compound is limited. Existing literature indicates that neodymium exposure can affect liver function and may have neurotoxic effects .
Antibacterial Properties
Recent studies have highlighted the antibacterial properties of tungsten oxide, which could extend to this compound due to the presence of tungsten. Tungsten oxide nanoparticles have demonstrated significant antibacterial activity against various bacterial strains, including Escherichia coli and Staphylococcus aureus. For instance, tungsten oxide nanodots showed a 70% reduction in E. coli viability after exposure to sunlight combined with the nanoparticles .
The mechanism behind this antibacterial effect is thought to involve the disruption of bacterial cell membranes, leading to cytoplasmic leakage and cell death . Although specific studies on this compound's antibacterial properties are scarce, it is plausible that the combination of neodymium and tungsten could enhance these effects.
Case Studies
- Pulmonary Toxicity Evaluation :
- Antibacterial Efficacy :
Research Findings Summary Table
Q & A
Basic Research Questions
Q. What are the optimal synthesis parameters for producing high-purity neodymium oxide (Nd₂O₃) via thermal decomposition?
- Methodological Answer : The synthesis of Nd₂O₃ via thermal decomposition of neodymium oxalate can be optimized using microwave calcination. Key parameters include:
- Temperature : 800–1000°C (to ensure complete decomposition).
- Heating Rate : Controlled ramp rates (e.g., 10°C/min) to avoid thermal gradients.
- Holding Time : 2–4 hours to achieve uniform particle morphology.
Characterization via thermogravimetry-differential scanning calorimetry (TG-DSC) confirms phase transitions, while X-ray diffraction (XRD) and scanning electron microscopy (SEM) validate crystallinity and particle uniformity. Microwave methods reduce thermal gradients compared to traditional calcination, yielding particles with narrow size distributions .
Q. How can researchers characterize the phase purity and morphology of neodymium oxide for advanced material studies?
- Methodological Answer : A multi-technique approach is recommended:
- XRD : To confirm hexagonal crystal structure (JCPDS 00-015-0847) and detect impurities.
- SEM/TEM : To analyze particle size (e.g., 15–80 nm for ultrafine powders) and morphology.
- BET Surface Area Analysis : To measure specific surface area (≥55 m²/g for ultrafine particles).
- Laser Particle Sizing : To assess size distribution.
Cross-referencing with standards (e.g., NIST databases) ensures accuracy .
Advanced Research Questions
Q. How can contradictions in carbochlorination conversion rates of neodymium oxide be analyzed statistically?
- Methodological Answer : Contradictions in conversion rates (e.g., 15.6–87.4% for Nd₂O₃) arise from process variability. Use a three-factor, two-level full factorial design with midpoint replication to model interactions between:
- Temperature (e.g., 500–700°C),
- Chlorine Concentration (e.g., 50–100 vol%),
- Carbon-to-Oxide Ratio (e.g., 0–100% excess).
Statistical tools like Design-Expert 8 can identify dominant factors (e.g., temperature) and optimize conditions. Residual analysis and ANOVA validate model reliability .
Q. What methodologies compare the environmental impact of primary production vs. recycling of neodymium oxide?
- Methodological Answer : Conduct a life cycle assessment (LCA) with the following steps:
- System Boundaries : Include mining, solvent extraction, calcination (primary) vs. manual dismantling/hydrogen processing (recycling).
- Key Metrics : Energy consumption, CO₂ emissions, and resource recovery rates.
- Data Sources : Experimental data (e.g., 92.3% conversion efficiency in primary routes) and literature (e.g., >90% recovery via manual recycling).
Results show recycling reduces environmental impact by 10× in energy use and emissions .
Q. How can electrolysis parameters be optimized for neodymium oxide reduction to metal?
- Methodological Answer : Key factors for electrolytic reduction of Nd₂O₃ in fluoride melts include:
- Electrolyte Composition : NdF₃-LiF mixtures (e.g., 75:25 mol%) for optimal ionic conductivity.
- Current Density : 0.5–1.0 A/cm² to balance deposition rate and dendritic growth.
- Anode Design : Graphite or composite anodes to minimize gas evolution (e.g., CO/CO₂).
Off-gas analysis (e.g., mass spectrometry) ensures process stability .
Q. What experimental approaches detect fission product migration in neodymium-containing nuclear fuels?
- Methodological Answer : Use shielded electron microprobe analysis to track axial migration of Nd in carbide fuels. Key steps:
Properties
CAS No. |
39361-84-7 |
---|---|
Molecular Formula |
NdOW |
Molecular Weight |
344.08 g/mol |
IUPAC Name |
neodymium;oxotungsten |
InChI |
InChI=1S/Nd.O.W |
InChI Key |
LMLOLRQHFCYMFP-UHFFFAOYSA-N |
Canonical SMILES |
O=[W].[Nd] |
Origin of Product |
United States |
Disclaimer and Information on In-Vitro Research Products
Please be aware that all articles and product information presented on BenchChem are intended solely for informational purposes. The products available for purchase on BenchChem are specifically designed for in-vitro studies, which are conducted outside of living organisms. In-vitro studies, derived from the Latin term "in glass," involve experiments performed in controlled laboratory settings using cells or tissues. It is important to note that these products are not categorized as medicines or drugs, and they have not received approval from the FDA for the prevention, treatment, or cure of any medical condition, ailment, or disease. We must emphasize that any form of bodily introduction of these products into humans or animals is strictly prohibited by law. It is essential to adhere to these guidelines to ensure compliance with legal and ethical standards in research and experimentation.