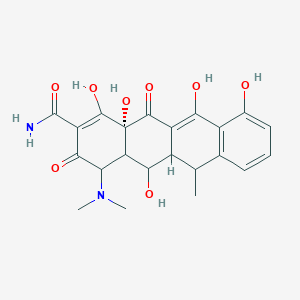
(12aR)-4-(dimethylamino)-1,5,10,11,12a-pentahydroxy-6-methyl-3,12-dioxo-4a,5,5a,6-tetrahydro-4H-tetracene-2-carboxamide
- Click on QUICK INQUIRY to receive a quote from our team of experts.
- With the quality product at a COMPETITIVE price, you can focus more on your research.
Overview
Description
(12aR)-4-(dimethylamino)-1,5,10,11,12a-pentahydroxy-6-methyl-3,12-dioxo-4a,5,5a,6-tetrahydro-4H-tetracene-2-carboxamide is a complex organic compound with a unique structure It is characterized by multiple hydroxyl groups, a dimethylamino group, and a carboxamide group
Preparation Methods
Synthetic Routes and Reaction Conditions
The synthesis of (12aR)-4-(dimethylamino)-1,5,10,11,12a-pentahydroxy-6-methyl-3,12-dioxo-4a,5,5a,6-tetrahydro-4H-tetracene-2-carboxamide involves multiple steps. The process typically starts with the preparation of the tetracene core, followed by the introduction of functional groups through various chemical reactions. Common reagents used in the synthesis include dimethylamine, hydroxylating agents, and carboxylating agents. The reaction conditions often involve controlled temperatures, pH levels, and the use of catalysts to ensure the desired product is obtained with high purity and yield .
Industrial Production Methods
In an industrial setting, the production of this compound may involve large-scale chemical reactors and continuous flow processes. The use of advanced purification techniques such as chromatography and crystallization is essential to achieve the required quality standards. The scalability of the synthesis process is crucial for its application in various industries .
Chemical Reactions Analysis
Types of Reactions
(12aR)-4-(dimethylamino)-1,5,10,11,12a-pentahydroxy-6-methyl-3,12-dioxo-4a,5,5a,6-tetrahydro-4H-tetracene-2-carboxamide undergoes several types of chemical reactions, including:
Oxidation: The hydroxyl groups can be oxidized to form ketones or aldehydes.
Reduction: The carbonyl groups can be reduced to hydroxyl groups.
Substitution: The dimethylamino group can be substituted with other functional groups under appropriate conditions
Common Reagents and Conditions
Common reagents used in these reactions include oxidizing agents like potassium permanganate, reducing agents like sodium borohydride, and various nucleophiles for substitution reactions. The reaction conditions vary depending on the desired transformation, but typically involve controlled temperatures, solvents, and catalysts .
Major Products
The major products formed from these reactions depend on the specific reagents and conditions used. For example, oxidation of the hydroxyl groups can lead to the formation of quinones, while reduction of the carbonyl groups can yield alcohols .
Scientific Research Applications
(12aR)-4-(dimethylamino)-1,5,10,11,12a-pentahydroxy-6-methyl-3,12-dioxo-4a,5,5a,6-tetrahydro-4H-tetracene-2-carboxamide has a wide range of scientific research applications:
Chemistry: It is used as a building block for the synthesis of more complex molecules and as a reagent in various organic reactions.
Biology: The compound’s unique structure makes it a valuable tool for studying enzyme interactions and protein binding.
Medicine: It has potential therapeutic applications due to its bioactive properties, including anti-inflammatory and anticancer activities.
Industry: The compound is used in the development of new materials and as a catalyst in chemical processes
Mechanism of Action
The mechanism of action of (12aR)-4-(dimethylamino)-1,5,10,11,12a-pentahydroxy-6-methyl-3,12-dioxo-4a,5,5a,6-tetrahydro-4H-tetracene-2-carboxamide involves its interaction with specific molecular targets and pathways. The compound can bind to enzymes and proteins, altering their activity and leading to various biological effects. The exact molecular targets and pathways depend on the specific application and context of use .
Comparison with Similar Compounds
Similar Compounds
- (4S,4aR,5S,5aR,6S,12aR)-4-(dimethylamino)-1,5,6,10,11,12a-hexahydroxy-6-methyl-3,12-dioxo-3,4,4a,5,5a,6,12,12a-octahydro-2-tetracenecarboxamide hydrochloride .
- (4S,4aS,5aR,12aR)-4,7-bis(dimethylamino)-1,10,11,12a-tetrahydroxy-3,12-dioxo-3,4,4a,5,5a,6,12,12a-octahydrotetracene-2-carbonitrile .
Uniqueness
The uniqueness of (12aR)-4-(dimethylamino)-1,5,10,11,12a-pentahydroxy-6-methyl-3,12-dioxo-4a,5,5a,6-tetrahydro-4H-tetracene-2-carboxamide lies in its specific arrangement of functional groups and stereochemistry, which confer distinct chemical and biological properties. This makes it a valuable compound for various research and industrial applications .
Biological Activity
The compound (12aR)-4-(dimethylamino)-1,5,10,11,12a-pentahydroxy-6-methyl-3,12-dioxo-4a,5,5a,6-tetrahydro-4H-tetracene-2-carboxamide is a complex organic molecule with significant biological activity, particularly as an antibiotic. Structurally related to doxycycline, it features multiple hydroxyl groups and a dimethylamino functional group, which enhance its solubility and bioavailability. The compound's molecular formula is C27H31N3O11 and it has a molecular weight of approximately 573.55 g/mol .
This compound primarily functions by inhibiting bacterial protein synthesis. It binds to the ribosomal subunit in bacteria, similar to other tetracycline derivatives. The presence of the pentahydroxy structure facilitates enhanced interaction with ribosomal RNA, leading to effective inhibition of protein synthesis in both Gram-positive and Gram-negative bacteria .
Key Mechanisms:
- Inhibition of Protein Synthesis: Binds to the ribosomal 30S subunit.
- Solubility Enhancement: Hydroxyl groups improve solubility in biological systems.
- Potential Anti-inflammatory Effects: May also exhibit anti-inflammatory properties, suggesting broader therapeutic applications beyond antibiotics .
Antimicrobial Spectrum
The compound has demonstrated effectiveness against various bacterial strains:
Bacterial Type | Activity |
---|---|
Gram-positive bacteria | Effective |
Gram-negative bacteria | Effective |
Mycoplasma | Effective |
Parasites | Potential activity noted |
Case Studies and Research Findings
- Antibiotic Efficacy : In a study comparing various tetracycline derivatives, (12aR)-4-(dimethylamino)-1,5,10,11,12a-pentahydroxy-6-methyl-3,12-dioxo was shown to have a lower minimum inhibitory concentration (MIC) against resistant strains of Staphylococcus aureus compared to traditional antibiotics .
- Anti-inflammatory Studies : Research indicates that this compound may reduce inflammation markers in vitro when tested on human cell lines exposed to inflammatory stimuli. This suggests potential applications in treating conditions like acne and respiratory infections .
- Pharmacokinetic Properties : Preliminary pharmacokinetic studies indicate that this compound has favorable absorption characteristics due to its structural features, allowing for effective systemic circulation post-administration .
The synthesis of (12aR)-4-(dimethylamino)-1,5,10,11,12a-pentahydroxy-6-methyl-3,12-dioxo typically involves multi-step organic reactions that include:
- Formation of the tetracene backbone.
- Introduction of hydroxyl groups through selective reduction reactions.
- Incorporation of the dimethylamino group via nucleophilic substitution.
Property | Value |
---|---|
Molecular Weight | 573.55 g/mol |
LogP | 0.271 |
PSA | 252.50 |
Properties
Molecular Formula |
C22H24N2O8 |
---|---|
Molecular Weight |
444.4 g/mol |
IUPAC Name |
(12aR)-4-(dimethylamino)-1,5,10,11,12a-pentahydroxy-6-methyl-3,12-dioxo-4a,5,5a,6-tetrahydro-4H-tetracene-2-carboxamide |
InChI |
InChI=1S/C22H24N2O8/c1-7-8-5-4-6-9(25)11(8)16(26)12-10(7)17(27)14-15(24(2)3)18(28)13(21(23)31)20(30)22(14,32)19(12)29/h4-7,10,14-15,17,25-27,30,32H,1-3H3,(H2,23,31)/t7?,10?,14?,15?,17?,22-/m0/s1 |
InChI Key |
SGKRLCUYIXIAHR-CHIQEMSVSA-N |
Isomeric SMILES |
CC1C2C(C3C(C(=O)C(=C([C@]3(C(=O)C2=C(C4=C1C=CC=C4O)O)O)O)C(=O)N)N(C)C)O |
Canonical SMILES |
CC1C2C(C3C(C(=O)C(=C(C3(C(=O)C2=C(C4=C1C=CC=C4O)O)O)O)C(=O)N)N(C)C)O |
Origin of Product |
United States |
Disclaimer and Information on In-Vitro Research Products
Please be aware that all articles and product information presented on BenchChem are intended solely for informational purposes. The products available for purchase on BenchChem are specifically designed for in-vitro studies, which are conducted outside of living organisms. In-vitro studies, derived from the Latin term "in glass," involve experiments performed in controlled laboratory settings using cells or tissues. It is important to note that these products are not categorized as medicines or drugs, and they have not received approval from the FDA for the prevention, treatment, or cure of any medical condition, ailment, or disease. We must emphasize that any form of bodily introduction of these products into humans or animals is strictly prohibited by law. It is essential to adhere to these guidelines to ensure compliance with legal and ethical standards in research and experimentation.