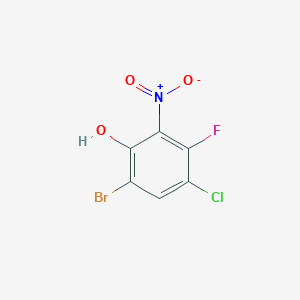
2-Bromo-4-chloro-5-fluoro-6-nitrophenol
- Click on QUICK INQUIRY to receive a quote from our team of experts.
- With the quality product at a COMPETITIVE price, you can focus more on your research.
Overview
Description
2-Bromo-4-chloro-5-fluoro-6-nitrophenol (C₆H₂BrClFNO₃) is a polyhalogenated nitrophenol derivative characterized by the presence of bromo (Br), chloro (Cl), fluoro (F), and nitro (NO₂) substituents on a phenolic ring. Structural analogs and computational predictions suggest that its reactivity and physicochemical properties are influenced by the electron-withdrawing effects of the nitro group and the halogen substituents, which enhance acidity and reduce solubility in nonpolar solvents .
Preparation Methods
Synthetic Routes and Reaction Conditions
The synthesis of 2-Bromo-4-chloro-5-fluoro-6-nitrophenol typically involves multi-step reactions starting from a phenol derivative. One common method includes the following steps:
Nitration: The phenol derivative undergoes nitration using a mixture of concentrated sulfuric acid and nitric acid to introduce the nitro group.
Halogenation: The nitrated phenol is then subjected to halogenation reactions. Bromination can be achieved using bromine in the presence of a catalyst such as iron(III) bromide. Chlorination and fluorination can be carried out using chlorine gas and a fluorinating agent like hydrogen fluoride, respectively.
Industrial Production Methods
Industrial production of this compound may involve similar synthetic routes but on a larger scale. The use of continuous flow reactors and optimized reaction conditions can enhance yield and purity. Safety measures are crucial due to the hazardous nature of some reagents.
Chemical Reactions Analysis
Types of Reactions
2-Bromo-4-chloro-5-fluoro-6-nitrophenol can undergo various chemical reactions, including:
Substitution Reactions: The halogen atoms (bromine, chlorine, fluorine) can be substituted by other groups under appropriate conditions.
Reduction Reactions: The nitro group can be reduced to an amino group using reducing agents like hydrogen gas in the presence of a catalyst.
Oxidation Reactions: The phenol group can be oxidized to a quinone derivative using oxidizing agents such as potassium permanganate.
Common Reagents and Conditions
Substitution: Nucleophiles like sodium methoxide can be used for substitution reactions.
Reduction: Catalytic hydrogenation or metal hydrides like sodium borohydride.
Oxidation: Potassium permanganate or chromium trioxide.
Major Products
Substitution: Formation of substituted phenol derivatives.
Reduction: Formation of 2-Bromo-4-chloro-5-fluoro-6-aminophenol.
Oxidation: Formation of quinone derivatives.
Scientific Research Applications
2-Bromo-4-chloro-5-fluoro-6-nitrophenol has several applications in scientific research:
Chemistry: Used as a building block for the synthesis of more complex molecules.
Biology: Investigated for its potential biological activities, including antimicrobial and anticancer properties.
Medicine: Explored for its potential use in drug development due to its unique chemical structure.
Industry: Utilized in the production of dyes, pigments, and other industrial chemicals.
Mechanism of Action
The mechanism of action of 2-Bromo-4-chloro-5-fluoro-6-nitrophenol depends on its specific application. For instance, its antimicrobial activity may involve the disruption of microbial cell membranes or inhibition of essential enzymes. In drug development, it may interact with specific molecular targets such as proteins or nucleic acids, affecting cellular pathways and processes.
Comparison with Similar Compounds
The following analysis compares 2-bromo-4-chloro-5-fluoro-6-nitrophenol with structurally related halogenated nitrophenols, focusing on substituent positions, physicochemical properties, and applications.
Table 1: Substituent Positions and Key Properties of Analogs
Key Comparisons:
Acidity: The nitro group at position 6 in this compound enhances acidity compared to analogs with nitro groups at positions 2 or 3. For example, 4-bromo-2-fluoro-6-nitrophenol (pKa ~3.2) is more acidic than 4-bromo-5-fluoro-2-nitrophenol (pKa ~4.1) due to stronger resonance stabilization of the deprotonated form . The chloro substituent at position 4 further increases acidity compared to non-chlorinated analogs (e.g., 2-bromo-3-fluoro-6-nitrophenol) by adding electron-withdrawing effects .
Solubility and Reactivity: Fluoro substituents at positions 5 or 2 reduce solubility in water but enhance compatibility with organic solvents. For instance, 2-bromo-3-fluoro-6-nitrophenol dissolves readily in ethanol, whereas 4-bromo-5-fluoro-2-nitrophenol requires polar aprotic solvents like DMF . The presence of chlorine in this compound may increase steric hindrance, slowing nucleophilic aromatic substitution compared to analogs without chloro groups .
Applications: Pharmaceuticals: Analogs like 4-bromo-5-fluoro-2-nitrophenol are used in photoaffinity probes due to nitro-group photolability . Agrochemicals: 2-Bromo-3-fluoro-6-nitrophenol serves as a precursor for herbicides, leveraging its stability under acidic conditions . Dyes: 4-Bromo-2-fluoro-6-nitrophenol’s strong acidity facilitates coupling reactions with diazonium salts to form azo dyes .
Biological Activity
2-Bromo-4-chloro-5-fluoro-6-nitrophenol (BCFNP) is a halogenated phenolic compound that has garnered attention for its diverse biological activities, particularly in antimicrobial and herbicidal applications. This article explores the compound's biological activity, synthesis methods, and its potential applications in agriculture and pharmaceuticals.
Chemical Structure and Properties
BCFNP has the molecular formula C6H3BrClFNO3 and a molecular weight of approximately 236.00 g/mol. Its structure features multiple halogen and nitro substituents on the aromatic ring, which significantly influence its reactivity and biological properties. The presence of bromine, chlorine, fluorine, and nitro groups enhances its efficacy against various pathogens and pests.
Antimicrobial Activity
BCFNP exhibits significant antimicrobial properties , making it effective against a range of bacterial strains. Research indicates that it can disrupt cellular processes in bacteria, leading to cell death. Its broad-spectrum antibacterial activity is attributed to its ability to interact with microbial cells and inhibit essential metabolic pathways.
Case Studies
- Antibacterial Efficacy : In a study evaluating the antibacterial effects of BCFNP, it was found to be effective against both Gram-positive and Gram-negative bacteria. The Minimum Inhibitory Concentration (MIC) values for various bacterial strains ranged from 10 to 50 µg/mL, demonstrating its potential as an antibacterial agent .
- Fungicidal Activity : Another study highlighted BCFNP's superior fungicidal activity compared to other phenolic compounds, such as 4-fluorophenol. It showed effective inhibition against phytopathogens like Gaeumannomyces graminis with an EC50 value of approximately 30.35 mg/L . This indicates its promise in controlling plant diseases.
Herbicidal Properties
BCFNP also demonstrates herbicidal activity , making it a candidate for agricultural applications. Its mechanism involves inhibiting weed growth through disruption of metabolic processes in target plants.
Experimental Findings
- In trials conducted on barnyard grass (Echinochloa crus-galli), BCFNP achieved a radicle inhibiting rate of 72.1% at a concentration of 80 mg/L, indicating strong potential as an herbicide .
- The compound was also effective against various weeds, showing promise for use in crop management strategies.
Synthesis Methods
The synthesis of BCFNP can be achieved through several methodologies, including:
- Nitration Method : A common approach involves dissolving 2-bromo-4-chlorophenol in chloroform and adding a nitrating mixture of sulfuric acid and nitric acid under controlled temperatures (40-80°C). This method allows for high yields and easier post-treatment processes .
- Alternative Synthetic Routes : Various patents outline alternative synthesis routes that emphasize different nitrating agents or solvents to optimize yield and purity .
Comparative Analysis with Related Compounds
The biological activity of BCFNP can be compared with structurally similar compounds:
Compound Name | Similarity | Key Features |
---|---|---|
1-Bromo-5-fluoro-2-methoxy-3-nitrobenzene | 0.90 | Contains a methoxy group; used in pharmaceuticals |
2-Bromo-3-fluoro-6-nitrophenol | 0.90 | Different substitution pattern; potential herbicide |
4-Bromo-2-fluoro-6-nitrophenol | 0.90 | Similar biological activity; used in research |
2,4-Dibromo-3-fluoro-6-nitrophenol | 0.89 | Increased bromination; enhanced biological effects |
6-Bromo-3-fluoro-2-nitrophenol | 0.89 | Different position of bromine; potential applications |
These compounds share structural similarities but exhibit distinct biological activities that can be leveraged for various applications in agriculture and medicine.
Q & A
Basic Research Questions
Q. What are the recommended methods for synthesizing 2-bromo-4-chloro-5-fluoro-6-nitrophenol, and how can reaction yields be optimized?
- Methodological Answer : Synthesis typically involves sequential halogenation and nitration of phenol derivatives. For example, bromination can be achieved using Br2 in acetic acid, followed by chlorination with SOCl2 or Cl2 under controlled temperatures (40–60°C). Fluorination may require HF or DAST, while nitration employs HNO3/H2SO4. Yield optimization requires monitoring reaction intermediates via TLC or HPLC and adjusting stoichiometry (e.g., excess halogenating agents for incomplete substitutions). Purity can be improved via recrystallization in ethanol/water mixtures .
Q. How should researchers characterize the structural and electronic properties of this compound?
- Methodological Answer : Use single-crystal X-ray diffraction (SC-XRD) to resolve the molecular geometry, as demonstrated for structurally similar bromo-chloro-phenol derivatives (e.g., bond angles and torsion angles) . Complement this with spectroscopic techniques:
- 1H/13C NMR : Assign peaks based on substituent-induced deshielding (e.g., nitro groups downfield at ~8–10 ppm for aromatic protons).
- FT-IR : Identify NO2 asymmetric stretching (~1520 cm−1) and phenolic O-H (~3200 cm−1).
- UV-Vis : Assess electronic transitions influenced by electron-withdrawing groups (e.g., λmax shifts due to nitro and halogens) .
Advanced Research Questions
Q. What experimental strategies can resolve contradictions in reported physicochemical properties (e.g., melting points, solubility)?
- Methodological Answer : Discrepancies often arise from impurities or polymorphic forms. To address this:
- Purification : Use column chromatography (silica gel, hexane/ethyl acetate gradient) followed by recrystallization.
- Thermal Analysis : Perform DSC/TGA to distinguish polymorphs (e.g., endothermic peaks for melting vs. decomposition).
- Solubility Studies : Use Hansen solubility parameters (HSPs) to correlate solvent polarity with solubility trends. For example, polar aprotic solvents like DMF may enhance solubility due to nitro/halogen interactions .
Q. How can computational modeling predict the reactivity of this compound in nucleophilic aromatic substitution (NAS) reactions?
- Methodological Answer : Apply density functional theory (DFT) to calculate partial charges and frontier molecular orbitals (FMOs). For example:
- Electrostatic Potential Maps : Identify electron-deficient aromatic carbons (activated by NO2 and halogens) as NAS sites.
- Activation Energy Barriers : Compare transition states for substitutions at Br vs. Cl positions using software like Gaussian or ORCA. Validate predictions with experimental kinetics (e.g., monitoring reaction rates via UV-Vis or 1H NMR) .
Q. What are the challenges in analyzing environmental degradation products of this compound, and how can they be mitigated?
- Methodological Answer : Degradation studies require:
- High-Resolution Mass Spectrometry (HRMS) : Detect low-concentration metabolites (e.g., dehalogenated or hydroxylated derivatives).
- Isotopic Labeling : Use 18O-labeled water or D2O to track hydrolysis pathways.
- Ecotoxicity Assays : Pair LC-MS data with Daphnia magna or algal growth inhibition tests to correlate degradation products with toxicity .
Q. Key Considerations for Experimental Design
Properties
Molecular Formula |
C6H2BrClFNO3 |
---|---|
Molecular Weight |
270.44 g/mol |
IUPAC Name |
6-bromo-4-chloro-3-fluoro-2-nitrophenol |
InChI |
InChI=1S/C6H2BrClFNO3/c7-2-1-3(8)4(9)5(6(2)11)10(12)13/h1,11H |
InChI Key |
UJSHMESTMITSJK-UHFFFAOYSA-N |
Canonical SMILES |
C1=C(C(=C(C(=C1Br)O)[N+](=O)[O-])F)Cl |
Origin of Product |
United States |
Disclaimer and Information on In-Vitro Research Products
Please be aware that all articles and product information presented on BenchChem are intended solely for informational purposes. The products available for purchase on BenchChem are specifically designed for in-vitro studies, which are conducted outside of living organisms. In-vitro studies, derived from the Latin term "in glass," involve experiments performed in controlled laboratory settings using cells or tissues. It is important to note that these products are not categorized as medicines or drugs, and they have not received approval from the FDA for the prevention, treatment, or cure of any medical condition, ailment, or disease. We must emphasize that any form of bodily introduction of these products into humans or animals is strictly prohibited by law. It is essential to adhere to these guidelines to ensure compliance with legal and ethical standards in research and experimentation.