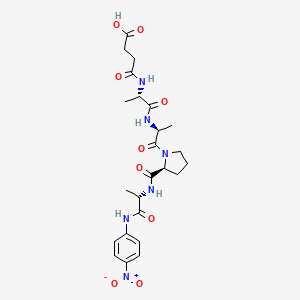
Suc-ala-ala-pro-ala-pna
Overview
Description
Suc-ala-ala-pro-ala-pna, also known as N-succinyl-alanyl-alanyl-prolyl-alanine p-nitroanilide, is a synthetic peptide substrate commonly used in enzymatic assays. This compound is particularly valuable for its role as a colorimetric substrate for elastase and other proteases. Upon enzymatic hydrolysis, it releases p-nitroaniline, which can be measured spectrophotometrically due to its yellow color .
Mechanism of Action
Target of Action
Suc-Ala-Ala-Pro-Ala-pNA is a peptide that primarily targets Prolyl Endopeptidase (PEP) . PEP is an enzyme that plays a crucial role in the metabolism of proline-containing peptides and is involved in the maturation and degradation of peptide hormones and neuropeptides.
Mode of Action
The compound acts as a substrate for PEP . It interacts with the active site of the enzyme, allowing the enzyme to bind and hydrolyze it. This interaction results in the release of p-nitroaniline , a yellow compound that can be quantified spectrophotometrically .
Biochemical Pathways
The primary biochemical pathway affected by this compound is the enzymatic activity of PEP. The hydrolysis of the compound by PEP leads to the release of p-nitroaniline , which can be used as a measure of PEP activity. This process plays a role in the metabolism of proline-containing peptides and the maturation and degradation of peptide hormones and neuropeptides.
Result of Action
The hydrolysis of this compound by PEP results in the release of p-nitroaniline . This release can be quantified spectrophotometrically, providing a measure of PEP activity. Therefore, the compound can be used to detect the activity and stability of PEP .
Action Environment
The action of this compound is influenced by environmental factors such as temperature and pH. For instance, the enzymatic activity of PEP, and thus the hydrolysis of the compound, can be measured at 30°C . Additionally, the compound’s solubility can be affected by the solvent used
Biochemical Analysis
Biochemical Properties
Suc-ala-ala-pro-ala-pna plays a crucial role in biochemical reactions as a substrate for several proteases, including chymotrypsin, cathepsin G, and peptidyl prolyl isomerase . The enzymatic cleavage of this compound results in the release of p-nitroaniline, which can be detected spectrophotometrically at 405 nm . This property makes it an excellent tool for studying enzyme kinetics and inhibitor screening.
Cellular Effects
This compound influences various cellular processes by serving as a substrate for proteases involved in cell signaling and metabolism . For instance, its interaction with chymotrypsin-like enzymes can affect protein degradation pathways, thereby influencing cellular homeostasis. Additionally, the cleavage of this compound by cathepsin G can impact immune cell function and inflammatory responses .
Molecular Mechanism
The molecular mechanism of this compound involves its binding to the active site of proteases, where it undergoes hydrolysis . This hydrolysis releases p-nitroaniline, which can be measured to determine enzyme activity. The specificity of this compound for certain proteases is due to its peptide sequence, which mimics natural substrates of these enzymes . This allows for precise measurement of protease activity and the study of enzyme-substrate interactions.
Temporal Effects in Laboratory Settings
In laboratory settings, the stability and degradation of this compound are critical factors that influence its effectiveness in assays . The compound is stable when stored desiccated at low temperatures, but its aqueous solutions should be prepared immediately before use to prevent spontaneous hydrolysis . Over time, the enzymatic activity measured using this compound can provide insights into the long-term effects of protease activity on cellular functions.
Dosage Effects in Animal Models
The effects of this compound in animal models vary with dosage . At optimal concentrations, it serves as an effective substrate for measuring protease activity without causing adverse effects. At high doses, there may be toxic effects or interference with normal cellular processes . These dosage-dependent effects are essential for determining the appropriate concentrations for experimental use.
Metabolic Pathways
This compound is involved in metabolic pathways related to protein degradation and turnover . It interacts with enzymes such as chymotrypsin and cathepsin G, which play roles in breaking down proteins into smaller peptides and amino acids. These interactions can influence metabolic flux and the levels of various metabolites within cells.
Preparation Methods
Synthetic Routes and Reaction Conditions
The synthesis of Suc-ala-ala-pro-ala-pna involves the stepwise assembly of the peptide chain using solid-phase peptide synthesis (SPPS). The process typically begins with the attachment of the C-terminal amino acid to a solid resin, followed by sequential addition of protected amino acids. Each amino acid is coupled using reagents such as dicyclohexylcarbodiimide (DCC) and N-hydroxybenzotriazole (HOBt). After the peptide chain is assembled, the protecting groups are removed, and the peptide is cleaved from the resin .
Industrial Production Methods
Industrial production of this compound follows similar principles as laboratory synthesis but on a larger scale. Automated peptide synthesizers are often employed to ensure high efficiency and reproducibility. The process involves rigorous purification steps, including high-performance liquid chromatography (HPLC), to achieve the desired purity levels .
Chemical Reactions Analysis
Types of Reactions
Suc-ala-ala-pro-ala-pna primarily undergoes hydrolysis reactions catalyzed by proteases. The hydrolysis of the peptide bond releases p-nitroaniline, which can be detected spectrophotometrically .
Common Reagents and Conditions
Reagents: Proteases such as elastase, chymotrypsin, and cathepsin G.
Conditions: The reactions are typically carried out in buffered aqueous solutions at specific pH values optimal for the enzyme activity.
Major Products
The major product of the hydrolysis reaction is p-nitroaniline, which exhibits a yellow color and can be quantified by measuring absorbance at 400-410 nm .
Scientific Research Applications
Suc-ala-ala-pro-ala-pna is widely used in scientific research for:
Enzyme Activity Assays: It serves as a substrate for measuring the activity of various proteases, including elastase, chymotrypsin, and cathepsin G.
Biological Studies: Used to study protease activity in different biological samples, such as blood, tissues, and cell lysates.
Medical Research: Helps in understanding the role of proteases in diseases and developing protease inhibitors as potential therapeutic agents.
Industrial Applications: Employed in quality control processes in the pharmaceutical and biotechnology industries to ensure the activity of protease enzymes.
Comparison with Similar Compounds
Similar Compounds
Suc-ala-ala-pro-phe-pna: Another peptide substrate used for similar enzymatic assays.
Suc-ala-pro-pna: Used to detect the activity of prolyl endopeptidase.
Uniqueness
Suc-ala-ala-pro-ala-pna is unique due to its specific sequence, which makes it a suitable substrate for a wide range of proteases. Its ability to release p-nitroaniline upon hydrolysis allows for easy and accurate measurement of enzyme activity .
Properties
IUPAC Name |
4-[[(2S)-1-[[(2S)-1-[(2S)-2-[[(2S)-1-(4-nitroanilino)-1-oxopropan-2-yl]carbamoyl]pyrrolidin-1-yl]-1-oxopropan-2-yl]amino]-1-oxopropan-2-yl]amino]-4-oxobutanoic acid | |
---|---|---|
Source | PubChem | |
URL | https://pubchem.ncbi.nlm.nih.gov | |
Description | Data deposited in or computed by PubChem | |
InChI |
InChI=1S/C24H32N6O9/c1-13(25-19(31)10-11-20(32)33)21(34)27-15(3)24(37)29-12-4-5-18(29)23(36)26-14(2)22(35)28-16-6-8-17(9-7-16)30(38)39/h6-9,13-15,18H,4-5,10-12H2,1-3H3,(H,25,31)(H,26,36)(H,27,34)(H,28,35)(H,32,33)/t13-,14-,15-,18-/m0/s1 | |
Source | PubChem | |
URL | https://pubchem.ncbi.nlm.nih.gov | |
Description | Data deposited in or computed by PubChem | |
InChI Key |
DKQHAQUESIKHGC-XSWJXKHESA-N | |
Source | PubChem | |
URL | https://pubchem.ncbi.nlm.nih.gov | |
Description | Data deposited in or computed by PubChem | |
Canonical SMILES |
CC(C(=O)NC(C)C(=O)N1CCCC1C(=O)NC(C)C(=O)NC2=CC=C(C=C2)[N+](=O)[O-])NC(=O)CCC(=O)O | |
Source | PubChem | |
URL | https://pubchem.ncbi.nlm.nih.gov | |
Description | Data deposited in or computed by PubChem | |
Isomeric SMILES |
C[C@@H](C(=O)N[C@@H](C)C(=O)N1CCC[C@H]1C(=O)N[C@@H](C)C(=O)NC2=CC=C(C=C2)[N+](=O)[O-])NC(=O)CCC(=O)O | |
Source | PubChem | |
URL | https://pubchem.ncbi.nlm.nih.gov | |
Description | Data deposited in or computed by PubChem | |
Molecular Formula |
C24H32N6O9 | |
Source | PubChem | |
URL | https://pubchem.ncbi.nlm.nih.gov | |
Description | Data deposited in or computed by PubChem | |
Molecular Weight |
548.5 g/mol | |
Source | PubChem | |
URL | https://pubchem.ncbi.nlm.nih.gov | |
Description | Data deposited in or computed by PubChem | |
Retrosynthesis Analysis
AI-Powered Synthesis Planning: Our tool employs the Template_relevance Pistachio, Template_relevance Bkms_metabolic, Template_relevance Pistachio_ringbreaker, Template_relevance Reaxys, Template_relevance Reaxys_biocatalysis model, leveraging a vast database of chemical reactions to predict feasible synthetic routes.
One-Step Synthesis Focus: Specifically designed for one-step synthesis, it provides concise and direct routes for your target compounds, streamlining the synthesis process.
Accurate Predictions: Utilizing the extensive PISTACHIO, BKMS_METABOLIC, PISTACHIO_RINGBREAKER, REAXYS, REAXYS_BIOCATALYSIS database, our tool offers high-accuracy predictions, reflecting the latest in chemical research and data.
Strategy Settings
Precursor scoring | Relevance Heuristic |
---|---|
Min. plausibility | 0.01 |
Model | Template_relevance |
Template Set | Pistachio/Bkms_metabolic/Pistachio_ringbreaker/Reaxys/Reaxys_biocatalysis |
Top-N result to add to graph | 6 |
Feasible Synthetic Routes
Q1: What is the significance of Suc-Ala-Ala-Pro-Ala-pNA in protease research?
A: this compound serves as a chromogenic substrate for specific serine proteases, primarily elastases. When hydrolyzed by these enzymes, the compound releases p-nitroaniline, a yellow-colored product. This color change allows for the easy detection and quantification of protease activity using spectrophotometry. [, ]
Q2: Are there any studies comparing the efficacy of this compound to other similar substrates?
A: Yes, research has demonstrated that Streptomyces elastase (SEL) hydrolyzes this compound with a 121-fold higher catalytic efficiency (kcat/Km) compared to another elastase substrate, Suc-Ala-Ala-Val-Ala-pNA. [] This highlights the importance of specific amino acid residues within the substrate sequence for optimal enzyme recognition and activity.
Disclaimer and Information on In-Vitro Research Products
Please be aware that all articles and product information presented on BenchChem are intended solely for informational purposes. The products available for purchase on BenchChem are specifically designed for in-vitro studies, which are conducted outside of living organisms. In-vitro studies, derived from the Latin term "in glass," involve experiments performed in controlled laboratory settings using cells or tissues. It is important to note that these products are not categorized as medicines or drugs, and they have not received approval from the FDA for the prevention, treatment, or cure of any medical condition, ailment, or disease. We must emphasize that any form of bodily introduction of these products into humans or animals is strictly prohibited by law. It is essential to adhere to these guidelines to ensure compliance with legal and ethical standards in research and experimentation.