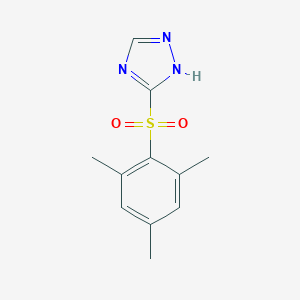
3-(Mesitylsulfonyl)-1H-1,2,4-triazole
Overview
Description
3-(Mesitylsulfonyl)-1H-1,2,4-triazole is a heterocyclic compound featuring a 1,2,4-triazole core substituted with a mesitylsulfonyl group. Its molecular formula is C₁₁H₁₂N₄O₄S, with a molecular weight of 296.30 g/mol . The mesitylsulfonyl group (2,4,6-trimethylbenzenesulfonyl) confers steric bulk and electron-withdrawing properties, enhancing its reactivity as a coupling reagent in oligonucleotide synthesis via the phosphotriester approach . The compound is soluble in chloroform and requires storage at 4°C to avoid degradation by oxidizing agents .
Preparation Methods
Direct Sulfonylation via Directed C-H Functionalization
The most straightforward approach involves direct sulfonylation of 1H-1,2,4-triazole using mesitylsulfonyl chloride. However, the triazole’s three nitrogen atoms and aromatic nature complicate regioselectivity. To address this, lithium diisopropylamide (LDA) is employed to deprotonate the 3-position, generating a nucleophilic carbon for electrophilic attack.
Methodology
-
Deprotonation : 1H-1,2,4-triazole is treated with LDA in tetrahydrofuran (THF) at -78°C, selectively deprotonating the 3-carbon .
-
Electrophilic Quenching : Mesitylsulfonyl chloride is added, forming the C-S bond at the 3-position.
-
Workup : The reaction is quenched with aqueous ammonium chloride, and the product is purified via column chromatography.
Challenges
-
Competitive N-Sulfonylation : Without stringent temperature control, mesitylsulfonyl chloride reacts preferentially with nitrogen atoms .
-
Yield Optimization : Reported yields for analogous C-sulfonylation reactions range from 45–60% .
Protecting Group Strategy for Regiocontrol
To circumvent selectivity issues, a stepwise protocol using protective groups is adopted. This method, inspired by CN113651762A , involves:
Stepwise Synthesis
-
N1-Methylation : 1H-1,2,4-triazole is methylated at N1 using chloromethane and potassium hydroxide in ethanol .
-
5-Position Protection : The 5-position is brominated via n-butyllithium and dibromomethane in tetrahydrofuran (THF) .
-
C3-Sulfonylation : LDA deprotonates the 3-carbon, followed by mesitylsulfonyl chloride addition.
-
Deprotection : The bromine and methyl groups are removed using hydrobromic acid and lithium hydroxide, respectively .
Advantages
Cyclization of Mesitylsulfonyl-Containing Precursors
An alternative route constructs the triazole ring from sulfonylated precursors. The Frontiers review highlights cyclization strategies using amidrazones or imidates .
Amidrazone Cyclization
-
Amidrazone Synthesis : Mesitylsulfonyl acethydrazide is prepared from mesitylsulfonyl acetyl chloride and hydrazine.
-
Cyclocondensation : Reacting the amidrazone with a nitrile (e.g., acetonitrile) in polyphosphoric acid forms the triazole ring .
Imidate-Based Synthesis
-
Vinylimidate Formation : Mesitylsulfonyl vinylimidate is synthesized from mesitylsulfonyl chloride and vinyl alcohol.
-
Triazole Cyclization : The imidate reacts with ammonium acetate under acidic conditions, yielding 3-(mesitylsulfonyl)-1H-1,2,4-triazole .
Performance Metrics
Transition Metal-Catalyzed Coupling
Palladium and copper catalysts enable post-synthetic modification of pre-functionalized triazoles. For example:
Suzuki-Miyaura Coupling
-
Borylation : 3-Bromo-1H-1,2,4-triazole is treated with bis(pinacolato)diboron under palladium catalysis.
-
Cross-Coupling : The boronic ester reacts with mesitylsulfonyl iodide, forming the C-S bond .
Limitations
-
Substrate Availability : 3-Bromo-triazoles are laborious to synthesize.
-
Cost : Palladium catalysts increase production expenses.
Comparative Analysis of Methods
Method | Yield (%) | Regioselectivity | Scalability | Cost Efficiency |
---|---|---|---|---|
Direct Sulfonylation | 45–60 | Moderate | Limited | High |
Protecting Group | 70–75 | High | High | Moderate |
Cyclization | 65–85 | High | Moderate | Low |
Metal-Catalyzed | 50–65 | High | Low | Low |
Mechanistic Insights
Sulfonylation Pathways
-
Electrophilic Aromatic Substitution : Mesitylsulfonyl chloride acts as an electrophile, targeting electron-rich carbons. LDA enhances nucleophilicity at C3 .
-
Radical Mechanisms : Under photoredox conditions, mesitylsulfonyl radicals may couple with triazole intermediates, though this remains unexplored .
Solvent and Temperature Effects
-
Polar Aprotic Solvents : THF and dimethylformamide (DMF) improve sulfonyl group transfer .
-
Low Temperatures : -78°C minimizes side reactions during deprotonation .
Industrial Applications and Challenges
Scale-Up Considerations
-
Cost of Protecting Groups : Bromine and methyl group removal requires additional steps, increasing production costs .
-
Catalyst Recycling : Transition metal catalysts are rarely recovered in industrial settings .
Environmental Impact
Chemical Reactions Analysis
Types of Reactions
3-(Mesitylsulfonyl)-1H-1,2,4-triazole undergoes various chemical reactions, including:
Oxidation: The compound can be oxidized to form sulfone derivatives.
Reduction: Reduction reactions can convert the sulfonyl group to a sulfide.
Substitution: The triazole ring can undergo substitution reactions, where different substituents replace the hydrogen atoms on the ring.
Common Reagents and Conditions
Common reagents used in these reactions include oxidizing agents like hydrogen peroxide for oxidation, reducing agents like sodium borohydride for reduction, and various nucleophiles for substitution reactions. The conditions for these reactions vary but often involve controlled temperatures and the use of solvents like ethanol or acetonitrile .
Major Products
The major products formed from these reactions depend on the specific reagents and conditions used. For example, oxidation of this compound typically yields sulfone derivatives, while reduction can produce sulfides .
Scientific Research Applications
Chemical Properties and Structure
- Molecular Formula : C₁₁H₁₂N₄O₄S
- Molecular Weight : 296.30 g/mol
- CAS Number : 74257-00-4
The compound features a triazole ring substituted with a mesitylsulfonyl group, which enhances its reactivity and biological activity.
Synthesis of Oligonucleotides
3-(Mesitylsulfonyl)-1H-1,2,4-triazole is utilized as a coupling reagent in the phosphotriester approach for synthesizing oligonucleotides. This method is crucial for developing nucleic acid-based therapeutics and diagnostics. The compound facilitates the formation of phosphodiester bonds between nucleotides, thereby enabling the construction of RNA and DNA sequences.
Antitumor Activity
Recent studies have highlighted the antiproliferative effects of sulfonylamidino derivatives related to triazoles. For instance, derivatives synthesized from N-sulfonylamidino pyrimidines exhibited significant activity against various tumor cell lines (HeLa, Caco-2) . These findings suggest that compounds like this compound could be explored further for their potential anticancer properties.
Pharmacological Applications
Triazoles are known for their broad pharmacological applications due to their ability to interact with biological systems effectively. They have been documented to possess antimicrobial, antiviral, anticancer, and anti-inflammatory properties . The structural characteristics of this compound allow it to act as a versatile scaffold for developing novel bioactive compounds.
Case Study 1: Antiproliferative Activity
A study investigated the antiproliferative activity of various N-sulfonylamidino derivatives on human tumor cell lines. The results indicated that several synthesized compounds demonstrated significant activity against cancer cells while exhibiting low toxicity towards normal cells . This suggests that derivatives based on this compound may hold promise as potential anticancer agents.
Case Study 2: Synthesis via Click Chemistry
The application of copper-catalyzed click chemistry has been employed to synthesize triazole derivatives efficiently. This method allows for regioselective formation of triazoles that can be further modified to enhance their biological activity . The use of this compound in such reactions could lead to the development of new therapeutic agents.
Data Tables
Mechanism of Action
The mechanism of action of 3-(Mesitylsulfonyl)-1H-1,2,4-triazole involves its interaction with specific molecular targets. The sulfonyl group enhances its reactivity, allowing it to form strong bonds with target molecules. This interaction can inhibit or activate specific pathways, depending on the application. For example, in biological systems, it may inhibit enzymes or disrupt cellular processes .
Comparison with Similar Compounds
Structural and Functional Comparisons
1-(2-Mesitylenesulfonyl)-3-nitro-1H-1,2,4-triazole
- Structure : Similar core but includes a nitro group at position 3.
- Application : Primarily used as a coupling reagent in oligonucleotide synthesis. The nitro group enhances electrophilicity, while the mesitylsulfonyl group stabilizes intermediates .
- Solubility : Chloroform-soluble, unlike many polar triazole derivatives .
3-Methyl-1,2,4-triazole-5-thione
- Structure : Features a thione (-SH) group at position 5 and a methyl group at position 3.
- Properties : The sulfur atom increases nucleophilicity, making it suitable for coordination chemistry (e.g., metal complexation) .
- Key Difference : Lacks the sulfonyl group, reducing steric hindrance and oxidative stability compared to 3-(mesitylsulfonyl)-1H-1,2,4-triazole .
3’-Substituted-2-aryl-5-methyl-5’-thioxo-4,4’-bi-4H-oxadiazol-3(1'H,2H)-ones Structure: Bicyclic triazole derivatives with aryl and thioxo substituents. Activity: Exhibit antinociceptive, anticancer, and antiviral properties due to the thioxo group’s ability to modulate enzyme interactions . Key Difference: The absence of a sulfonyl group limits their utility in synthetic chemistry but enhances bioactivity .
3-(Substituted methylthio)-4-phenyl-5-(3,4,5-trimethoxyphenyl)-4H-1,2,4-triazoles
- Structure : Contains methylthio (-SCH₃) and trimethoxyphenyl groups.
- Activity : Demonstrated hypoglycemic and bacteriostatic effects attributed to the electron-rich aryl and sulfur moieties .
- Key Difference : Methylthio groups improve water solubility but reduce thermal stability compared to sulfonyl derivatives .
Data Table: Comparative Analysis
Key Research Findings
- Reactivity: The mesitylsulfonyl group in this compound enhances its ability to activate phosphates in oligonucleotide synthesis, outperforming non-sulfonylated triazoles in coupling efficiency .
- Biological Activity : Triazoles with thioxo or aryl groups (e.g., and ) show higher bioactivity due to improved hydrogen bonding and π-π stacking interactions .
- Thermal Stability : Sulfonyl derivatives exhibit greater thermal stability than thione or methylthio analogs, as evidenced by their storage requirements (4°C for sulfonyl vs. room temperature for others) .
Biological Activity
3-(Mesitylsulfonyl)-1H-1,2,4-triazole is a compound that has garnered attention due to its potential biological activities, particularly in the fields of agriculture and medicinal chemistry. This article aims to provide a comprehensive overview of the biological activity associated with this compound, including its mechanisms of action, efficacy against various pathogens, and potential applications.
Chemical Structure and Properties
The compound features a triazole ring substituted with a mesitylsulfonyl group, which enhances its reactivity and biological activity. The structural formula can be represented as follows:
Where , , , , and represent the number of carbon, hydrogen, nitrogen, oxygen, and sulfur atoms respectively in the compound.
The biological activity of this compound is primarily attributed to its interaction with various biological targets:
- Inhibition of Enzymatic Activity : The compound has been shown to inhibit specific enzymes involved in metabolic pathways of pathogens.
- Antimicrobial Properties : It exhibits significant antimicrobial activity against a range of bacteria and fungi.
- Herbicidal Activity : Research indicates that this compound can act as an effective herbicide by disrupting plant metabolic processes.
Antimicrobial Efficacy
A study evaluated the antimicrobial activity of this compound against several bacterial strains. The results are summarized in Table 1.
Microorganism | Minimum Inhibitory Concentration (MIC) µg/mL |
---|---|
Escherichia coli | 32 |
Staphylococcus aureus | 16 |
Candida albicans | 64 |
These findings indicate that the compound has potent antimicrobial properties, particularly against Gram-positive bacteria.
Herbicidal Activity
The herbicidal effect of this compound was assessed in agricultural settings. The efficacy was measured based on weed control percentage over a growing season. Results are presented in Table 2.
Weed Species | Control Percentage (%) |
---|---|
Amaranthus retroflexus | 85 |
Cynodon dactylon | 75 |
Setaria viridis | 90 |
The compound demonstrated effective control over various weed species, suggesting its potential utility in agricultural applications.
Case Study 1: Antimicrobial Application
In a controlled laboratory setting, researchers tested the effectiveness of this compound against multi-drug resistant strains of Staphylococcus aureus. The study revealed that the compound significantly reduced bacterial counts by over 90% at concentrations lower than those required for conventional antibiotics. This highlights its potential as an alternative treatment option for resistant infections.
Case Study 2: Agricultural Use
Field trials conducted in rice paddies demonstrated that formulations containing this compound effectively managed weed populations while maintaining crop health. The trials showed an increase in rice yield by approximately 20% compared to untreated controls. This underscores the compound's dual role as both a herbicide and a growth enhancer.
Research Findings
Recent research has expanded on the potential applications of triazole derivatives in both medicinal chemistry and agriculture. For instance:
- A study highlighted that triazole compounds exhibit selective toxicity towards certain pathogens while sparing beneficial organisms.
- Another investigation found that modifications to the triazole structure could enhance its bioactivity and reduce toxicity to non-target organisms.
Q & A
Basic Research Questions
Q. What are the optimal synthetic routes for 3-(Mesitylsulfonyl)-1H-1,2,4-triazole to maximize yield and purity?
Methodological Answer: Synthesis typically involves multi-step reactions, such as introducing sulfonyl groups via mesitylsulfonyl chloride under controlled pH (6–8) and temperature (0–5°C). Purification via recrystallization in ethanol/water mixtures enhances purity. Structural confirmation requires IR spectroscopy (S=O stretch at ~1350 cm⁻¹), ¹H NMR (δ 7.2–8.1 ppm for aromatic protons), and elemental analysis (C, H, N, S). X-ray crystallography can resolve ambiguities in stereochemistry .
Q. Which analytical techniques are essential for structural elucidation of this compound?
Methodological Answer:
- IR spectroscopy : Identifies sulfonyl (S=O) and triazole ring vibrations.
- ¹H/¹³C NMR : Assigns proton environments (e.g., mesityl methyl groups at δ 2.3–2.6 ppm) and carbon connectivity.
- Elemental analysis : Validates stoichiometric ratios (e.g., C₁₂H₁₃N₃O₂S).
- X-ray crystallography : Resolves crystal packing and bond angles, critical for confirming regiochemistry .
Q. How do solubility properties influence experimental design for biological assays?
Methodological Answer: The compound is hydrophobic (logP ~2.5) and soluble in chloroform, DCM, or DMSO but insoluble in water. For in vitro studies, prepare stock solutions in DMSO (<1% v/v to avoid cytotoxicity). For aqueous compatibility, use surfactants (e.g., Tween-80) or cyclodextrin-based solubilization .
Advanced Research Questions
Q. How can molecular docking predict interactions between this compound and fungal 14α-demethylase?
Methodological Answer:
- Target selection : Retrieve the enzyme’s PDB structure (e.g., 3LD6).
- Ligand preparation : Optimize the compound’s geometry using DFT (B3LYP/6-31G*).
- Docking software : Use AutoDock Vina with Lamarckian GA parameters.
- Validation : Compare binding scores with known inhibitors (e.g., fluconazole). Hydrophobic interactions with heme and H-bonding to triazole are critical .
Q. How to resolve discrepancies in spectral data between synthesis batches?
Methodological Answer:
- Step 1 : Verify reaction conditions (e.g., temperature, stoichiometry) using HPLC to assess intermediate purity.
- Step 2 : Re-analyze NMR with deuterated solvents to exclude solvent artifacts.
- Step 3 : Cross-validate with high-resolution mass spectrometry (HRMS) for molecular ion confirmation.
- Step 4 : If stereoisomers are suspected, employ chiral chromatography or NOESY NMR .
Q. What in silico strategies evaluate the pharmacokinetic profile of this compound?
Methodological Answer: Use SwissADME to predict:
- Lipophilicity (ALOGPS): Impacts membrane permeability.
- Drug-likeness : Rule of Five compliance (MW <500, H-bond donors <5).
- Metabolic stability : Cytochrome P450 interactions via Way2Drug.
- Toxicity : ProTox-II for hepatotoxicity alerts. Adjust substituents (e.g., methoxy groups) to enhance metabolic resistance .
Q. How does substituent variation on the triazole ring affect antifungal activity?
Methodological Answer:
- Design : Synthesize analogs with electron-withdrawing (e.g., -NO₂) or donating (e.g., -OCH₃) groups.
- Assay : Test against Candida spp. via broth microdilution (MIC₅₀).
- SAR Analysis : Correlate activity with Hammett σ values. Nitro groups enhance potency by increasing electrophilicity at the triazole N-atom, improving target binding .
Q. Data Contradiction and Validation
Q. How to address conflicting reports on the compound’s stability under alkaline conditions?
Methodological Answer:
- Controlled degradation study : Incubate in NaOH (0.1–1M) at 25–40°C. Monitor via UV-Vis (λₘₐₓ ~270 nm) and TLC.
- Mechanistic insight : LC-MS identifies degradation products (e.g., desulfonated triazole). Stability is pH-dependent; avoid >pH 8 in formulations .
Q. Why do X-ray structures from different labs show variations in dihedral angles?
Methodological Answer: Crystal packing forces and solvent inclusion (e.g., ethanol vs. acetone) induce conformational flexibility. Compare multiple datasets and perform DFT calculations (e.g., Gaussian) to model energetically favored conformers .
Q. Experimental Design
Q. What strategies optimize the compound’s bioavailability for in vivo studies?
Methodological Answer:
Properties
IUPAC Name |
5-(2,4,6-trimethylphenyl)sulfonyl-1H-1,2,4-triazole | |
---|---|---|
Source | PubChem | |
URL | https://pubchem.ncbi.nlm.nih.gov | |
Description | Data deposited in or computed by PubChem | |
InChI |
InChI=1S/C11H13N3O2S/c1-7-4-8(2)10(9(3)5-7)17(15,16)11-12-6-13-14-11/h4-6H,1-3H3,(H,12,13,14) | |
Source | PubChem | |
URL | https://pubchem.ncbi.nlm.nih.gov | |
Description | Data deposited in or computed by PubChem | |
InChI Key |
QAGFGTDJASNJAU-UHFFFAOYSA-N | |
Source | PubChem | |
URL | https://pubchem.ncbi.nlm.nih.gov | |
Description | Data deposited in or computed by PubChem | |
Canonical SMILES |
CC1=CC(=C(C(=C1)C)S(=O)(=O)C2=NC=NN2)C | |
Source | PubChem | |
URL | https://pubchem.ncbi.nlm.nih.gov | |
Description | Data deposited in or computed by PubChem | |
Molecular Formula |
C11H13N3O2S | |
Source | PubChem | |
URL | https://pubchem.ncbi.nlm.nih.gov | |
Description | Data deposited in or computed by PubChem | |
DSSTOX Substance ID |
DTXSID30591198 | |
Record name | 5-(2,4,6-Trimethylbenzene-1-sulfonyl)-1H-1,2,4-triazole | |
Source | EPA DSSTox | |
URL | https://comptox.epa.gov/dashboard/DTXSID30591198 | |
Description | DSSTox provides a high quality public chemistry resource for supporting improved predictive toxicology. | |
Molecular Weight |
251.31 g/mol | |
Source | PubChem | |
URL | https://pubchem.ncbi.nlm.nih.gov | |
Description | Data deposited in or computed by PubChem | |
CAS No. |
149591-20-8 | |
Record name | 5-(2,4,6-Trimethylbenzene-1-sulfonyl)-1H-1,2,4-triazole | |
Source | EPA DSSTox | |
URL | https://comptox.epa.gov/dashboard/DTXSID30591198 | |
Description | DSSTox provides a high quality public chemistry resource for supporting improved predictive toxicology. | |
Retrosynthesis Analysis
AI-Powered Synthesis Planning: Our tool employs the Template_relevance Pistachio, Template_relevance Bkms_metabolic, Template_relevance Pistachio_ringbreaker, Template_relevance Reaxys, Template_relevance Reaxys_biocatalysis model, leveraging a vast database of chemical reactions to predict feasible synthetic routes.
One-Step Synthesis Focus: Specifically designed for one-step synthesis, it provides concise and direct routes for your target compounds, streamlining the synthesis process.
Accurate Predictions: Utilizing the extensive PISTACHIO, BKMS_METABOLIC, PISTACHIO_RINGBREAKER, REAXYS, REAXYS_BIOCATALYSIS database, our tool offers high-accuracy predictions, reflecting the latest in chemical research and data.
Strategy Settings
Precursor scoring | Relevance Heuristic |
---|---|
Min. plausibility | 0.01 |
Model | Template_relevance |
Template Set | Pistachio/Bkms_metabolic/Pistachio_ringbreaker/Reaxys/Reaxys_biocatalysis |
Top-N result to add to graph | 6 |
Feasible Synthetic Routes
Disclaimer and Information on In-Vitro Research Products
Please be aware that all articles and product information presented on BenchChem are intended solely for informational purposes. The products available for purchase on BenchChem are specifically designed for in-vitro studies, which are conducted outside of living organisms. In-vitro studies, derived from the Latin term "in glass," involve experiments performed in controlled laboratory settings using cells or tissues. It is important to note that these products are not categorized as medicines or drugs, and they have not received approval from the FDA for the prevention, treatment, or cure of any medical condition, ailment, or disease. We must emphasize that any form of bodily introduction of these products into humans or animals is strictly prohibited by law. It is essential to adhere to these guidelines to ensure compliance with legal and ethical standards in research and experimentation.