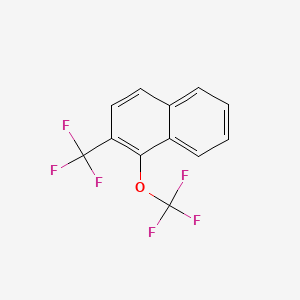
1-(Trifluoromethoxy)-2-(trifluoromethyl)naphthalene
- Click on QUICK INQUIRY to receive a quote from our team of experts.
- With the quality product at a COMPETITIVE price, you can focus more on your research.
Overview
Description
1-(Trifluoromethoxy)-2-(trifluoromethyl)naphthalene is an organic compound characterized by the presence of both trifluoromethoxy and trifluoromethyl groups attached to a naphthalene ring
Preparation Methods
The synthesis of 1-(trifluoromethoxy)-2-(trifluoromethyl)naphthalene typically involves the introduction of trifluoromethoxy and trifluoromethyl groups onto a naphthalene ring. One common synthetic route involves the reaction of naphthalene derivatives with trifluoromethylating and trifluoromethoxylating agents under specific conditions. For instance, the use of trifluoromethyl iodide (CF3I) and trifluoromethoxy iodide (CF3OI) in the presence of a suitable catalyst can facilitate the formation of the desired compound .
Industrial production methods may involve similar synthetic routes but on a larger scale, with optimizations for yield, purity, and cost-effectiveness. The choice of reagents, catalysts, and reaction conditions can vary depending on the specific requirements of the production process.
Chemical Reactions Analysis
1-(Trifluoromethoxy)-2-(trifluoromethyl)naphthalene can undergo various chemical reactions, including:
Oxidation: The compound can be oxidized using strong oxidizing agents, leading to the formation of naphthoquinone derivatives.
Reduction: Reduction reactions can be carried out using reducing agents such as lithium aluminum hydride (LiAlH4), resulting in the formation of partially or fully reduced naphthalene derivatives.
Substitution: The trifluoromethoxy and trifluoromethyl groups can participate in nucleophilic substitution reactions, where nucleophiles replace these groups under appropriate conditions.
Common reagents used in these reactions include oxidizing agents like potassium permanganate (KMnO4), reducing agents like LiAlH4, and nucleophiles such as amines and thiols. The major products formed depend on the specific reaction conditions and the nature of the reagents used .
Scientific Research Applications
1-(Trifluoromethoxy)-2-(trifluoromethyl)naphthalene has several scientific research applications:
Chemistry: It serves as a building block for the synthesis of more complex fluorinated organic compounds, which are valuable in various chemical research and industrial applications.
Biology: The compound’s unique fluorinated structure makes it a useful probe in biological studies, particularly in understanding the interactions of fluorinated molecules with biological systems.
Medicine: Fluorinated compounds, including this compound, are explored for their potential therapeutic properties, such as enzyme inhibition and receptor modulation.
Mechanism of Action
The mechanism of action of 1-(trifluoromethoxy)-2-(trifluoromethyl)naphthalene involves its interaction with molecular targets through its trifluoromethoxy and trifluoromethyl groups. These groups can influence the compound’s electronic properties, making it a potent modulator of enzyme activity and receptor binding. The pathways involved may include inhibition of specific enzymes or alteration of receptor signaling, depending on the biological context .
Comparison with Similar Compounds
1-(Trifluoromethoxy)-2-(trifluoromethyl)naphthalene can be compared with other similar compounds, such as:
1-(Trifluoromethoxy)-naphthalene: Lacks the trifluoromethyl group, resulting in different chemical and biological properties.
2-(Trifluoromethyl)naphthalene: Lacks the trifluoromethoxy group, leading to variations in reactivity and applications.
Trifluoromethyl-substituted benzenes: These compounds have similar trifluoromethyl groups but differ in their aromatic ring structure, affecting their overall properties and uses.
The uniqueness of this compound lies in the combined presence of both trifluoromethoxy and trifluoromethyl groups on a naphthalene ring, which imparts distinct chemical and biological characteristics.
Properties
Molecular Formula |
C12H6F6O |
---|---|
Molecular Weight |
280.16 g/mol |
IUPAC Name |
1-(trifluoromethoxy)-2-(trifluoromethyl)naphthalene |
InChI |
InChI=1S/C12H6F6O/c13-11(14,15)9-6-5-7-3-1-2-4-8(7)10(9)19-12(16,17)18/h1-6H |
InChI Key |
LIFALNNOHQNUCI-UHFFFAOYSA-N |
Canonical SMILES |
C1=CC=C2C(=C1)C=CC(=C2OC(F)(F)F)C(F)(F)F |
Origin of Product |
United States |
Disclaimer and Information on In-Vitro Research Products
Please be aware that all articles and product information presented on BenchChem are intended solely for informational purposes. The products available for purchase on BenchChem are specifically designed for in-vitro studies, which are conducted outside of living organisms. In-vitro studies, derived from the Latin term "in glass," involve experiments performed in controlled laboratory settings using cells or tissues. It is important to note that these products are not categorized as medicines or drugs, and they have not received approval from the FDA for the prevention, treatment, or cure of any medical condition, ailment, or disease. We must emphasize that any form of bodily introduction of these products into humans or animals is strictly prohibited by law. It is essential to adhere to these guidelines to ensure compliance with legal and ethical standards in research and experimentation.