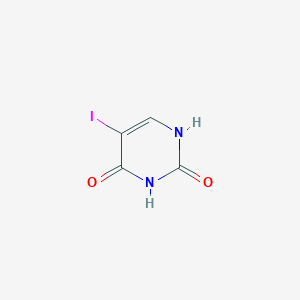
5-Iodouracil
Overview
Description
5-Iodouracil is a halogenated pyrimidine that can be used in nucleoprotein photo-crosslinking via RNA substitution . It is used in thymidine phosphorylase targeted imaging and therapy . DNA N-glycosylase MED1 exhibited higher preference for 5-Iodouracil and halogenated bases over non-halogenated ones .
Synthesis Analysis
The reactions of 5-iodouracil with sterically hindered RBr such as R = t-C4H9 and 1-adamantyl (1-Adm) failed to give the products under the same conditions or when the reaction was carried out in N,N-dimethylformamide containing triethylamine at 140 °C for 10 h .Molecular Structure Analysis
5-Iodouracil has a molecular weight of 237.9833 and a chemical formula of C4H3IN2O2 . It belongs to the class of organic compounds known as halopyrimidines, which are aromatic compounds containing a halogen atom linked to a pyrimidine ring .Chemical Reactions Analysis
5-Iodouracil can be integrated into DNA and acts as a UV sensitive chromophore suitable for probing DNA structure and DNA-protein interactions based on the photochemical reactions of 5-IU .Physical And Chemical Properties Analysis
5-Iodouracil is a white to light yellow fluffy powder . It has a molecular weight of 237.98 and an empirical formula of C4H3IN2O2 .Scientific Research Applications
Antimicrobial Agent
5-Iodouracil has been studied for its potential as an antimicrobial agent. The synthesis of N-substituted 5-Iodouracils has shown that certain analogs can inhibit the growth of various microorganisms. For instance, compounds with N1-substitution displayed inhibition against strains like Branhamella catarrhalis, Neisseria mucosa, and Streptococcus pyogenes .
Anticancer Activity
The anticancer properties of 5-Iodouracil derivatives have been a subject of interest due to their ability to inhibit the growth of cancer cells. Specifically, N1,N3-dicyclohexylmethyl analogs have demonstrated potent anticancer activity, with one analog exhibiting an IC50 of 16.5 μg/mL against HepG2 liver cancer cells .
Photocrosslinking in Nucleoprotein Complexes
5-Iodouracil is an effective chromophore for photocrosslinking nucleoprotein complexes, which is a technique used to study the interactions between nucleic acids and proteins. This compound achieves high yield photocrosslinking, often three to five times higher than that achieved with 5-bromouracil, due to its absorption at longer wavelengths .
Antiviral Research
Research has indicated that uracils possessing halogens at the 5-position, such as 5-Iodouracil, have antiviral activities. This makes 5-Iodouracil a candidate for further exploration in the development of antiviral drugs .
Biochemical Studies
5-Iodouracil can be used in biochemical studies to investigate the mechanisms of action of nucleic acids and their interactions with other biomolecules. Its incorporation into RNA or DNA allows for detailed analysis of nucleoprotein complexes and their functions .
Medicinal Chemistry
In medicinal chemistry, 5-Iodouracil serves as a building block for the synthesis of more complex molecules with potential therapeutic applications. Its reactivity and halogen presence make it a versatile compound for creating a variety of bioactive molecules .
Safety and Hazards
5-Iodouracil is harmful if swallowed, in contact with skin, or if inhaled. It causes skin irritation, serious eye irritation, and may cause respiratory irritation . It is recommended to avoid breathing dust/fume/gas/mist/vapors/spray, and to wear protective gloves/protective clothing/eye protection/face protection .
Relevant Papers There are several papers that discuss 5-Iodouracil. For example, one paper discusses the mechanistic studies of the 5-Iodouracil chromophore relevant to its use in nucleoprotein photo-crosslinking . Another paper discusses the high yield photocrosslinking of a 5-Iodocytidine (IC) substituted RNA .
Mechanism of Action
Target of Action
The primary target of 5-Iodouracil is Dihydropyrimidine dehydrogenase [NADP (+)] . This enzyme is involved in pyrimidine base degradation and catalyzes the reduction of uracil and thymine .
Mode of Action
5-Iodouracil acts as an inhibitor of its target enzyme. The three-dimensional structure of the ternary complex enzyme-NADPH-5-iodouracil supports the proposal that this compound acts as a mechanism-based inhibitor, covalently modifying the active-site residue Cys-671, resulting in S-(hexahydro-2,4-dioxo-5-pyrimidinyl)cysteine .
Result of Action
The molecular and cellular effects of 5-Iodouracil’s action involve high yield photocrosslinking of nucleoprotein complexes . The crosslinking yields are often three to five times higher than those achieved with 5-bromouracil . The technique is primarily selective for aromatic amino acid residues; consequently, success in crosslinking depends upon proximity of a U or T of the nucleic acid to an aromatic ring of the protein .
Action Environment
The action of 5-Iodouracil is influenced by environmental factors such as light. The highest yields of photocrosslinking are achieved by irradiating with a helium cadmium laser emitting at 325 nm . Useful yields can also be obtained with a 312 nm transilluminator .
properties
IUPAC Name |
5-iodo-1H-pyrimidine-2,4-dione | |
---|---|---|
Source | PubChem | |
URL | https://pubchem.ncbi.nlm.nih.gov | |
Description | Data deposited in or computed by PubChem | |
InChI |
InChI=1S/C4H3IN2O2/c5-2-1-6-4(9)7-3(2)8/h1H,(H2,6,7,8,9) | |
Source | PubChem | |
URL | https://pubchem.ncbi.nlm.nih.gov | |
Description | Data deposited in or computed by PubChem | |
InChI Key |
KSNXJLQDQOIRIP-UHFFFAOYSA-N | |
Source | PubChem | |
URL | https://pubchem.ncbi.nlm.nih.gov | |
Description | Data deposited in or computed by PubChem | |
Canonical SMILES |
C1=C(C(=O)NC(=O)N1)I | |
Source | PubChem | |
URL | https://pubchem.ncbi.nlm.nih.gov | |
Description | Data deposited in or computed by PubChem | |
Molecular Formula |
C4H3IN2O2 | |
Source | PubChem | |
URL | https://pubchem.ncbi.nlm.nih.gov | |
Description | Data deposited in or computed by PubChem | |
DSSTOX Substance ID |
DTXSID3061009 | |
Record name | 2,4(1H,3H)-Pyrimidinedione, 5-iodo- | |
Source | EPA DSSTox | |
URL | https://comptox.epa.gov/dashboard/DTXSID3061009 | |
Description | DSSTox provides a high quality public chemistry resource for supporting improved predictive toxicology. | |
Molecular Weight |
237.98 g/mol | |
Source | PubChem | |
URL | https://pubchem.ncbi.nlm.nih.gov | |
Description | Data deposited in or computed by PubChem | |
Product Name |
5-Iodouracil | |
CAS RN |
696-07-1 | |
Record name | 5-Iodouracil | |
Source | CAS Common Chemistry | |
URL | https://commonchemistry.cas.org/detail?cas_rn=696-07-1 | |
Description | CAS Common Chemistry is an open community resource for accessing chemical information. Nearly 500,000 chemical substances from CAS REGISTRY cover areas of community interest, including common and frequently regulated chemicals, and those relevant to high school and undergraduate chemistry classes. This chemical information, curated by our expert scientists, is provided in alignment with our mission as a division of the American Chemical Society. | |
Explanation | The data from CAS Common Chemistry is provided under a CC-BY-NC 4.0 license, unless otherwise stated. | |
Record name | 5-Iodouracil | |
Source | ChemIDplus | |
URL | https://pubchem.ncbi.nlm.nih.gov/substance/?source=chemidplus&sourceid=0000696071 | |
Description | ChemIDplus is a free, web search system that provides access to the structure and nomenclature authority files used for the identification of chemical substances cited in National Library of Medicine (NLM) databases, including the TOXNET system. | |
Record name | 5-Iodouracil | |
Source | DrugBank | |
URL | https://www.drugbank.ca/drugs/DB03554 | |
Description | The DrugBank database is a unique bioinformatics and cheminformatics resource that combines detailed drug (i.e. chemical, pharmacological and pharmaceutical) data with comprehensive drug target (i.e. sequence, structure, and pathway) information. | |
Explanation | Creative Common's Attribution-NonCommercial 4.0 International License (http://creativecommons.org/licenses/by-nc/4.0/legalcode) | |
Record name | 5-Iodouracil | |
Source | DTP/NCI | |
URL | https://dtp.cancer.gov/dtpstandard/servlet/dwindex?searchtype=NSC&outputformat=html&searchlist=57848 | |
Description | The NCI Development Therapeutics Program (DTP) provides services and resources to the academic and private-sector research communities worldwide to facilitate the discovery and development of new cancer therapeutic agents. | |
Explanation | Unless otherwise indicated, all text within NCI products is free of copyright and may be reused without our permission. Credit the National Cancer Institute as the source. | |
Record name | 2,4(1H,3H)-Pyrimidinedione, 5-iodo- | |
Source | EPA Chemicals under the TSCA | |
URL | https://www.epa.gov/chemicals-under-tsca | |
Description | EPA Chemicals under the Toxic Substances Control Act (TSCA) collection contains information on chemicals and their regulations under TSCA, including non-confidential content from the TSCA Chemical Substance Inventory and Chemical Data Reporting. | |
Record name | 2,4(1H,3H)-Pyrimidinedione, 5-iodo- | |
Source | EPA DSSTox | |
URL | https://comptox.epa.gov/dashboard/DTXSID3061009 | |
Description | DSSTox provides a high quality public chemistry resource for supporting improved predictive toxicology. | |
Record name | 5-iodouracil | |
Source | European Chemicals Agency (ECHA) | |
URL | https://echa.europa.eu/substance-information/-/substanceinfo/100.010.718 | |
Description | The European Chemicals Agency (ECHA) is an agency of the European Union which is the driving force among regulatory authorities in implementing the EU's groundbreaking chemicals legislation for the benefit of human health and the environment as well as for innovation and competitiveness. | |
Explanation | Use of the information, documents and data from the ECHA website is subject to the terms and conditions of this Legal Notice, and subject to other binding limitations provided for under applicable law, the information, documents and data made available on the ECHA website may be reproduced, distributed and/or used, totally or in part, for non-commercial purposes provided that ECHA is acknowledged as the source: "Source: European Chemicals Agency, http://echa.europa.eu/". Such acknowledgement must be included in each copy of the material. ECHA permits and encourages organisations and individuals to create links to the ECHA website under the following cumulative conditions: Links can only be made to webpages that provide a link to the Legal Notice page. | |
Record name | 5-IODOURACIL | |
Source | FDA Global Substance Registration System (GSRS) | |
URL | https://gsrs.ncats.nih.gov/ginas/app/beta/substances/H59BRK500M | |
Description | The FDA Global Substance Registration System (GSRS) enables the efficient and accurate exchange of information on what substances are in regulated products. Instead of relying on names, which vary across regulatory domains, countries, and regions, the GSRS knowledge base makes it possible for substances to be defined by standardized, scientific descriptions. | |
Explanation | Unless otherwise noted, the contents of the FDA website (www.fda.gov), both text and graphics, are not copyrighted. They are in the public domain and may be republished, reprinted and otherwise used freely by anyone without the need to obtain permission from FDA. Credit to the U.S. Food and Drug Administration as the source is appreciated but not required. | |
Synthesis routes and methods I
Procedure details
Synthesis routes and methods II
Procedure details
Retrosynthesis Analysis
AI-Powered Synthesis Planning: Our tool employs the Template_relevance Pistachio, Template_relevance Bkms_metabolic, Template_relevance Pistachio_ringbreaker, Template_relevance Reaxys, Template_relevance Reaxys_biocatalysis model, leveraging a vast database of chemical reactions to predict feasible synthetic routes.
One-Step Synthesis Focus: Specifically designed for one-step synthesis, it provides concise and direct routes for your target compounds, streamlining the synthesis process.
Accurate Predictions: Utilizing the extensive PISTACHIO, BKMS_METABOLIC, PISTACHIO_RINGBREAKER, REAXYS, REAXYS_BIOCATALYSIS database, our tool offers high-accuracy predictions, reflecting the latest in chemical research and data.
Strategy Settings
Precursor scoring | Relevance Heuristic |
---|---|
Min. plausibility | 0.01 |
Model | Template_relevance |
Template Set | Pistachio/Bkms_metabolic/Pistachio_ringbreaker/Reaxys/Reaxys_biocatalysis |
Top-N result to add to graph | 6 |
Feasible Synthetic Routes
Disclaimer and Information on In-Vitro Research Products
Please be aware that all articles and product information presented on BenchChem are intended solely for informational purposes. The products available for purchase on BenchChem are specifically designed for in-vitro studies, which are conducted outside of living organisms. In-vitro studies, derived from the Latin term "in glass," involve experiments performed in controlled laboratory settings using cells or tissues. It is important to note that these products are not categorized as medicines or drugs, and they have not received approval from the FDA for the prevention, treatment, or cure of any medical condition, ailment, or disease. We must emphasize that any form of bodily introduction of these products into humans or animals is strictly prohibited by law. It is essential to adhere to these guidelines to ensure compliance with legal and ethical standards in research and experimentation.