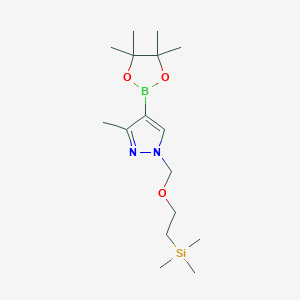
3-methyl-4-(4,4,5,5-tetramethyl-1,3,2-dioxaborolan-2-yl)-1-((2-(trimethylsilyl)ethoxy)methyl)-1H-pyrazole
Overview
Description
The compound “3-methyl-4-(4,4,5,5-tetramethyl-1,3,2-dioxaborolan-2-yl)-1-((2-(trimethylsilyl)ethoxy)methyl)-1H-pyrazole” is a complex organic molecule. It contains a pyrazole ring, which is a type of heterocyclic aromatic organic compound .
Molecular Structure Analysis
The molecular structure of this compound is complex, with a pyrazole ring and a dioxaborolane ring, both of which are types of heterocyclic aromatic organic compounds . It also contains a trimethylsilyl group, which is commonly used in organic chemistry as a protecting group .Scientific Research Applications
Synthesis and Characterization
Synthesis and Characterization Techniques : Studies like those by Liao et al. (2022) and Huang et al. (2021) focus on synthesizing similar compounds and characterizing their structures using techniques like FT-IR, NMR, MS spectroscopy, and X-ray diffraction. These methods are crucial in confirming the molecular structures and understanding the physicochemical properties of such compounds (Liao et al., 2022); (Huang et al., 2021).
Density Functional Theory (DFT) Studies : Research like that by Yang et al. (2021) involves using DFT to calculate molecular structures and comparing these results with X-ray diffraction values, providing insights into the molecular structure and conformation (Yang et al., 2021).
Applications in Organic Synthesis
Intermediate in Organic Synthesis : Compounds like 3-methyl-4-(4,4,5,5-tetramethyl-1,3,2-dioxaborolan-2-yl)-1-((2-(trimethylsilyl)ethoxy)methyl)-1H-pyrazole often serve as intermediates in the synthesis of biologically active compounds, as demonstrated in works like that of Kong et al. (2016) (Kong et al., 2016).
Ligand Synthesis for Metal Complexes : These compounds can also be used in synthesizing ligands for metal complexes, which have diverse applications in areas such as catalysis and materials science, as shown in research by Budzisz et al. (2004) (Budzisz et al., 2004).
Potential in Material Science
Nanoparticle and Polymer Research : Studies like those by Fischer et al. (2013) demonstrate the use of similar compounds in the creation of nanoparticles and polymers, which can have significant implications in material science, particularly in the fields of photoluminescence and electroluminescence (Fischer et al., 2013).
Synthesis of Colored Polymers : Research by Welterlich et al. (2012) illustrates how these compounds can contribute to the synthesis of deeply colored polymers, which have potential applications in coatings, inks, and display technologies (Welterlich et al., 2012).
Safety And Hazards
Future Directions
properties
IUPAC Name |
trimethyl-[2-[[3-methyl-4-(4,4,5,5-tetramethyl-1,3,2-dioxaborolan-2-yl)pyrazol-1-yl]methoxy]ethyl]silane | |
---|---|---|
Source | PubChem | |
URL | https://pubchem.ncbi.nlm.nih.gov | |
Description | Data deposited in or computed by PubChem | |
InChI |
InChI=1S/C16H31BN2O3Si/c1-13-14(17-21-15(2,3)16(4,5)22-17)11-19(18-13)12-20-9-10-23(6,7)8/h11H,9-10,12H2,1-8H3 | |
Source | PubChem | |
URL | https://pubchem.ncbi.nlm.nih.gov | |
Description | Data deposited in or computed by PubChem | |
InChI Key |
KERWYBKIAHNWRQ-UHFFFAOYSA-N | |
Source | PubChem | |
URL | https://pubchem.ncbi.nlm.nih.gov | |
Description | Data deposited in or computed by PubChem | |
Canonical SMILES |
B1(OC(C(O1)(C)C)(C)C)C2=CN(N=C2C)COCC[Si](C)(C)C | |
Source | PubChem | |
URL | https://pubchem.ncbi.nlm.nih.gov | |
Description | Data deposited in or computed by PubChem | |
Molecular Formula |
C16H31BN2O3Si | |
Source | PubChem | |
URL | https://pubchem.ncbi.nlm.nih.gov | |
Description | Data deposited in or computed by PubChem | |
Molecular Weight |
338.3 g/mol | |
Source | PubChem | |
URL | https://pubchem.ncbi.nlm.nih.gov | |
Description | Data deposited in or computed by PubChem | |
Product Name |
3-methyl-4-(4,4,5,5-tetramethyl-1,3,2-dioxaborolan-2-yl)-1-((2-(trimethylsilyl)ethoxy)methyl)-1H-pyrazole |
Retrosynthesis Analysis
AI-Powered Synthesis Planning: Our tool employs the Template_relevance Pistachio, Template_relevance Bkms_metabolic, Template_relevance Pistachio_ringbreaker, Template_relevance Reaxys, Template_relevance Reaxys_biocatalysis model, leveraging a vast database of chemical reactions to predict feasible synthetic routes.
One-Step Synthesis Focus: Specifically designed for one-step synthesis, it provides concise and direct routes for your target compounds, streamlining the synthesis process.
Accurate Predictions: Utilizing the extensive PISTACHIO, BKMS_METABOLIC, PISTACHIO_RINGBREAKER, REAXYS, REAXYS_BIOCATALYSIS database, our tool offers high-accuracy predictions, reflecting the latest in chemical research and data.
Strategy Settings
Precursor scoring | Relevance Heuristic |
---|---|
Min. plausibility | 0.01 |
Model | Template_relevance |
Template Set | Pistachio/Bkms_metabolic/Pistachio_ringbreaker/Reaxys/Reaxys_biocatalysis |
Top-N result to add to graph | 6 |
Feasible Synthetic Routes
Disclaimer and Information on In-Vitro Research Products
Please be aware that all articles and product information presented on BenchChem are intended solely for informational purposes. The products available for purchase on BenchChem are specifically designed for in-vitro studies, which are conducted outside of living organisms. In-vitro studies, derived from the Latin term "in glass," involve experiments performed in controlled laboratory settings using cells or tissues. It is important to note that these products are not categorized as medicines or drugs, and they have not received approval from the FDA for the prevention, treatment, or cure of any medical condition, ailment, or disease. We must emphasize that any form of bodily introduction of these products into humans or animals is strictly prohibited by law. It is essential to adhere to these guidelines to ensure compliance with legal and ethical standards in research and experimentation.