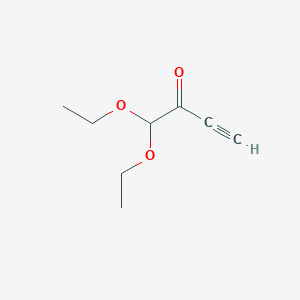
1,1-Diethoxybut-3-yn-2-one
Overview
Description
1,1-Diethoxybut-3-yn-2-one is an organic compound characterized by the presence of both ethoxy and alkyne functional groups
Mechanism of Action
Target of Action
1,1-Diethoxybut-3-yn-2-one is a conjugated terminal acetylenic ketone . Its primary targets are nitrogen and oxygen nucleophiles . These nucleophiles play a crucial role in the Michael addition reactions .
Mode of Action
The compound interacts with its targets through Michael addition reactions . In most cases, the predominant reaction is monoaddition . This leads to the formation of the corresponding β-substituted α,β-unsaturated ketones . The stereochemistry of the resulting compounds depends on the nature of the nucleophile .
Biochemical Pathways
The compound is involved in the Diels-Alder reactions . It reacts with a few conjugated dienes at room temperature and above . The expected cyclohexa-1,4-diene adducts are formed, but they are unstable and aromatize to the corresponding benzene derivatives .
Pharmacokinetics
The compound’s reactivity and the rate of its reactions can influence its absorption, distribution, metabolism, and excretion (adme) properties .
Result of Action
The result of the compound’s action is the formation of β-substituted α,β-unsaturated ketones . These are formed in a stereospecific fashion . In some cases, the Michael-addition products are unstable and undergo secondary reactions to form heterocyclic compounds .
Action Environment
The action of this compound is influenced by environmental factors such as temperature and the presence of other reactants . For instance, the compound reacts with conjugated dienes at room temperature and above . The rate of reaction is also influenced by the concentration of the reactants .
Biochemical Analysis
Biochemical Properties
1,1-Diethoxybut-3-yn-2-one plays a significant role in biochemical reactions, particularly in the formation of Michael adducts with nitrogen and oxygen nucleophiles . These reactions often result in the formation of β-substituted α,β-unsaturated ketones. The compound interacts with various enzymes and proteins, facilitating the addition of nucleophiles to the carbon-carbon triple bond. This interaction is crucial for the compound’s reactivity and its ability to participate in complex biochemical pathways.
Cellular Effects
This compound has been shown to influence cellular processes by interacting with cellular nucleophiles. This interaction can affect cell signaling pathways, gene expression, and cellular metabolism. The compound’s ability to form adducts with nucleophiles can lead to changes in cellular function, potentially impacting cell growth and differentiation. Studies have indicated that this compound can modulate the activity of specific enzymes involved in metabolic pathways, thereby influencing overall cellular metabolism .
Molecular Mechanism
The molecular mechanism of this compound involves its reactivity with nucleophiles, leading to the formation of Michael adducts. This process typically results in the addition of nucleophiles to the α,β-unsaturated ketone, forming stable adducts. The compound’s ability to undergo these reactions is attributed to the electron-withdrawing nature of the ethoxy groups, which enhance the electrophilicity of the carbon-carbon triple bond. This increased reactivity allows this compound to participate in various biochemical reactions, including enzyme inhibition or activation and changes in gene expression .
Temporal Effects in Laboratory Settings
In laboratory settings, the effects of this compound can change over time due to its stability and potential degradation. The compound is relatively stable under standard laboratory conditions, but prolonged exposure to air and moisture can lead to hydrolysis and degradation. Studies have shown that the compound’s reactivity can decrease over time, impacting its effectiveness in biochemical assays. Long-term effects on cellular function have been observed in both in vitro and in vivo studies, with changes in enzyme activity and gene expression noted over extended periods .
Dosage Effects in Animal Models
The effects of this compound vary with different dosages in animal models. At low doses, the compound has been shown to modulate enzyme activity and influence metabolic pathways without causing significant toxicity. At higher doses, toxic effects have been observed, including cellular damage and adverse effects on organ function. Threshold effects have been identified, indicating that there is a specific dosage range within which the compound can exert its biochemical effects without causing harm .
Metabolic Pathways
This compound is involved in various metabolic pathways, primarily through its interactions with enzymes and cofactors. The compound can be metabolized by enzymes such as cytochrome P450, leading to the formation of reactive intermediates. These intermediates can further react with cellular nucleophiles, influencing metabolic flux and altering metabolite levels. The compound’s involvement in these pathways highlights its potential impact on cellular metabolism and its utility in biochemical research .
Transport and Distribution
Within cells and tissues, this compound is transported and distributed through interactions with transporters and binding proteins. These interactions facilitate the compound’s localization to specific cellular compartments, where it can exert its biochemical effects. The compound’s distribution is influenced by its chemical properties, including its solubility and reactivity with cellular components .
Subcellular Localization
The subcellular localization of this compound is determined by its interactions with targeting signals and post-translational modifications. These interactions direct the compound to specific organelles, such as the mitochondria or endoplasmic reticulum, where it can participate in biochemical reactions. The compound’s localization can impact its activity and function, influencing cellular processes and metabolic pathways .
Preparation Methods
Synthetic Routes and Reaction Conditions: 1,1-Diethoxybut-3-yn-2-one can be synthesized through the reaction of ethyl vinyl ether with propargyl alcohol under acidic conditions. The reaction typically involves the use of a strong acid catalyst, such as sulfuric acid, to facilitate the formation of the desired product .
Industrial Production Methods: In an industrial setting, the synthesis of this compound may involve continuous flow reactors to optimize yield and efficiency. The process would likely include steps for purification, such as distillation or recrystallization, to ensure the high purity of the final product .
Chemical Reactions Analysis
Types of Reactions: 1,1-Diethoxybut-3-yn-2-one undergoes various types of chemical reactions, including:
Diels-Alder Reactions: This compound acts as a dienophile in Diels-Alder reactions, forming cyclohexadiene derivatives when reacted with conjugated dienes.
Cycloaddition Reactions: It participates in [3+2] cycloaddition reactions with azides to form triazoles.
Common Reagents and Conditions:
Diels-Alder Reactions: Typically conducted under reflux conditions with a large excess of diene and solvents like toluene.
Cycloaddition Reactions: Often carried out in the presence of copper(I) salts as catalysts, with solvents such as dichloromethane.
Major Products:
Diels-Alder Reactions: The major products are cyclohexadiene derivatives, which can further aromatize to form benzene derivatives.
Cycloaddition Reactions: The primary products are 1,2,3-triazoles, which are valuable intermediates in organic synthesis.
Scientific Research Applications
1,1-Diethoxybut-3-yn-2-one has several applications in scientific research:
Organic Synthesis: It is used as a building block in the synthesis of complex organic molecules, including natural products and pharmaceuticals.
Materials Science: The compound’s unique reactivity makes it useful in the development of new materials with specific properties, such as polymers and nanomaterials.
Medicinal Chemistry: Its derivatives are explored for potential therapeutic applications, including as intermediates in drug synthesis.
Comparison with Similar Compounds
But-3-yn-2-one: Lacks the ethoxy groups, making it less reactive in certain cycloaddition reactions.
1,1-Diethoxybut-2-yn-3-one: Similar structure but different positioning of the ethoxy groups, leading to different reactivity patterns.
Uniqueness: 1,1-Diethoxybut-3-yn-2-one is unique due to the presence of both ethoxy and alkyne groups, which confer distinct reactivity and make it a versatile intermediate in organic synthesis .
Properties
IUPAC Name |
1,1-diethoxybut-3-yn-2-one | |
---|---|---|
Source | PubChem | |
URL | https://pubchem.ncbi.nlm.nih.gov | |
Description | Data deposited in or computed by PubChem | |
InChI |
InChI=1S/C8H12O3/c1-4-7(9)8(10-5-2)11-6-3/h1,8H,5-6H2,2-3H3 | |
Source | PubChem | |
URL | https://pubchem.ncbi.nlm.nih.gov | |
Description | Data deposited in or computed by PubChem | |
InChI Key |
XGLPCTZTKHBEGQ-UHFFFAOYSA-N | |
Source | PubChem | |
URL | https://pubchem.ncbi.nlm.nih.gov | |
Description | Data deposited in or computed by PubChem | |
Canonical SMILES |
CCOC(C(=O)C#C)OCC | |
Source | PubChem | |
URL | https://pubchem.ncbi.nlm.nih.gov | |
Description | Data deposited in or computed by PubChem | |
Molecular Formula |
C8H12O3 | |
Source | PubChem | |
URL | https://pubchem.ncbi.nlm.nih.gov | |
Description | Data deposited in or computed by PubChem | |
Molecular Weight |
156.18 g/mol | |
Source | PubChem | |
URL | https://pubchem.ncbi.nlm.nih.gov | |
Description | Data deposited in or computed by PubChem | |
Retrosynthesis Analysis
AI-Powered Synthesis Planning: Our tool employs the Template_relevance Pistachio, Template_relevance Bkms_metabolic, Template_relevance Pistachio_ringbreaker, Template_relevance Reaxys, Template_relevance Reaxys_biocatalysis model, leveraging a vast database of chemical reactions to predict feasible synthetic routes.
One-Step Synthesis Focus: Specifically designed for one-step synthesis, it provides concise and direct routes for your target compounds, streamlining the synthesis process.
Accurate Predictions: Utilizing the extensive PISTACHIO, BKMS_METABOLIC, PISTACHIO_RINGBREAKER, REAXYS, REAXYS_BIOCATALYSIS database, our tool offers high-accuracy predictions, reflecting the latest in chemical research and data.
Strategy Settings
Precursor scoring | Relevance Heuristic |
---|---|
Min. plausibility | 0.01 |
Model | Template_relevance |
Template Set | Pistachio/Bkms_metabolic/Pistachio_ringbreaker/Reaxys/Reaxys_biocatalysis |
Top-N result to add to graph | 6 |
Feasible Synthetic Routes
Q1: How does 1,1-Diethoxybut-3-yn-2-one interact with different nucleophiles? What determines the stereochemistry of the products?
A1: this compound, a conjugated terminal acetylenic ketone, readily undergoes Michael addition reactions with various nitrogen and oxygen nucleophiles. [] This reaction primarily yields monoaddition products, forming β-substituted α,β-unsaturated ketones with specific stereochemistry. Interestingly, the nucleophile's nature dictates the product's stereochemistry. Ammonia and primary amines typically lead to the formation of Z-alkenones. In contrast, secondary amines and alcohols favor the formation of E-alkenones. [] This selectivity highlights the influence of steric and electronic factors on the reaction pathway.
Q2: Can this compound participate in Diels-Alder reactions? What factors influence its reactivity in these reactions?
A2: While this compound itself readily participates in Diels-Alder reactions with conjugated dienes, its corresponding alcohol derivatives (1,1-diethoxy-5-hydroxyalk-3-yn-2-ones) show limited reactivity. [] This difference suggests that the presence of the free hydroxyl group hinders the Diels-Alder process. The reactions typically require elevated temperatures and often result in the formation of benzene derivatives as the final products, likely due to the instability of the initial cyclohexa-1,4-diene adducts. []
Disclaimer and Information on In-Vitro Research Products
Please be aware that all articles and product information presented on BenchChem are intended solely for informational purposes. The products available for purchase on BenchChem are specifically designed for in-vitro studies, which are conducted outside of living organisms. In-vitro studies, derived from the Latin term "in glass," involve experiments performed in controlled laboratory settings using cells or tissues. It is important to note that these products are not categorized as medicines or drugs, and they have not received approval from the FDA for the prevention, treatment, or cure of any medical condition, ailment, or disease. We must emphasize that any form of bodily introduction of these products into humans or animals is strictly prohibited by law. It is essential to adhere to these guidelines to ensure compliance with legal and ethical standards in research and experimentation.