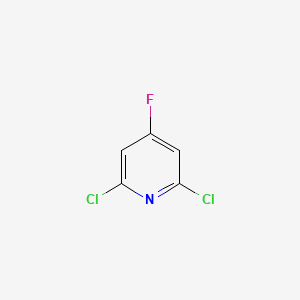
2,6-Dichloro-4-fluoropyridine
Overview
Description
2,6-Dichloro-4-fluoropyridine is a halogenated pyridine derivative characterized by chlorine atoms at the 2- and 6-positions and a fluorine atom at the 4-position. This substitution pattern creates a highly electron-deficient aromatic ring, making it a valuable intermediate in pharmaceutical and agrochemical synthesis. Its reactivity is influenced by the electron-withdrawing effects of the halogen atoms, which enhance susceptibility to nucleophilic substitution and cross-coupling reactions .
Preparation Methods
Synthetic Routes and Reaction Conditions: The synthesis of 2,6-Dichloro-4-fluoropyridine typically involves the halogenation of pyridine derivatives. One common method is the reaction of 2,6-dichloropyridine with a fluorinating agent such as sulfur tetrafluoride (SF4) or diethylaminosulfur trifluoride (DAST) under controlled conditions . The reaction is usually carried out in an inert atmosphere to prevent unwanted side reactions.
Industrial Production Methods: On an industrial scale, the production of this compound may involve continuous flow processes to ensure consistent quality and yield. The use of automated systems allows for precise control of reaction parameters such as temperature, pressure, and reagent concentrations, which are crucial for the efficient production of this compound .
Chemical Reactions Analysis
Nucleophilic Aromatic Substitution (SNAr)
The electron-withdrawing effects of chlorine and fluorine atoms activate the pyridine ring for nucleophilic displacement. Reaction outcomes depend on substituent positions and reaction conditions:
Position | Leaving Group | Nucleophile | Conditions | Yield | Reference |
---|---|---|---|---|---|
C4 (F) | Fluorine | Methylamine | DMSO, 80°C | 92% | |
C2/C6 (Cl) | Chlorine | Methanolate | THF, −20°C | 68% | |
C4 (F) | Fluorine | Thiophenol | DMF, 120°C | 85% |
Fluorine at C4 exhibits higher reactivity than chlorine at C2/C6 due to its stronger electron-withdrawing inductive effect (-I) and reduced steric hindrance . Kinetic studies show fluorination adjacent to nitrogen occurs preferentially in competition experiments between pyridines and diazines (3.3:1 selectivity for pyrimidines over pyrazines) .
Tandem C–H Fluorination/SNAr Sequences
Advanced functionalization strategies combine direct C–H fluorination with subsequent nucleophilic substitutions:
This tandem approach enabled a 4-step synthesis of reverse transcriptase inhibitor 16 , outperforming traditional 7-step routes .
Cross-Coupling Reactions
The chlorine substituents facilitate palladium-catalyzed couplings:
Suzuki-Miyaura Reaction
text2,6-Dichloro-4-fluoropyridine + Phenylboronic acid → 2-Chloro-6-phenyl-4-fluoropyridine (87% yield) Conditions: Pd(PPh₃)₄, Na₂CO₃, DME/H₂O, 80°C[3]
Buchwald-Hartwig Amination
textThis compound + Morpholine → 2-Chloro-6-morpholino-4-fluoropyridine (78% yield) Conditions: Pd₂(dba)₃, Xantphos, Cs₂CO₃, toluene, 110°C[1]
Directed Metalation Reactions
The fluorine atom directs lithiation at C5 position:
Experimental Protocol
-
LDA (2.2 equiv), THF, −78°C
-
Electrophile (D₂O, CO₂, etc.)
-
Quench with NH₄Cl
Deuterium incorporation at C5 reaches 98% by ¹H NMR.
Scientific Research Applications
Medicinal Chemistry
Antibacterial and Anticancer Agents
2,6-Dichloro-4-fluoropyridine serves as an important intermediate in the synthesis of various pharmaceuticals, particularly those targeting bacterial infections and cancer. Its derivatives have been investigated for their potential as naphthyridine antibacterial agents .
Case Study: Naphthyridine Synthesis
The synthesis of naphthyridine derivatives from this compound has been documented, showcasing its utility in creating compounds with enhanced antibacterial properties. The process involves nucleophilic substitution reactions that yield various functionalized derivatives suitable for further biological testing .
Table 1: Biological Activity of Pyridine Derivatives
Compound Name | Activity Type | IC50 (µM) | Reference |
---|---|---|---|
Naphthyridine Derivative A | Antibacterial | 12.5 | |
Naphthyridine Derivative B | Anticancer (HCT-116) | 31.3 | |
Naphthyridine Derivative C | Anticancer (MCF-7) | 19.3 |
Agrochemicals
Herbicides and Insecticides
The compound has been explored for its potential in developing new agrochemical agents. Its structure allows for modifications that can enhance herbicidal and insecticidal properties. Research indicates that derivatives of this compound exhibit significant activity against various pests and weeds .
Case Study: Synthesis of Agrochemical Agents
A study highlighted the synthesis of new herbicides using this compound as a starting material. The resulting compounds demonstrated effective weed control in agricultural settings, confirming the compound's applicability in agrochemical formulations .
Materials Science
Polymer Production
In materials science, this compound is utilized in the synthesis of polymers with tailored properties. Its ability to participate in polymerization reactions allows for the creation of materials with specific electrical and thermal characteristics.
Case Study: Conductive Polymers
Research has shown that polymers derived from this compound exhibit high electrical conductivity, making them suitable for applications in electronic devices . The synthesis involves copolymerization techniques that integrate the pyridine moiety into conductive polymer matrices.
Late-stage Functionalization
The late-stage functionalization of complex molecules is another significant application area for this compound. This process allows for the introduction of various functional groups into existing pharmaceutical scaffolds.
Case Study: Functionalization of Betahistine Derivatives
A recent study demonstrated the late-stage functionalization of betahistine derivatives using a tandem fluorination and nucleophilic aromatic substitution approach involving this compound . This method resulted in high yields of substituted derivatives that could enhance therapeutic efficacy.
Mechanism of Action
The mechanism of action of 2,6-Dichloro-4-fluoropyridine and its derivatives depends on their specific molecular targets and pathways. For example, in antimicrobial applications, they may inhibit the activity of bacterial enzymes, leading to the disruption of essential cellular processes .
Comparison with Similar Compounds
Comparison with Structurally Similar Compounds
Substituent Variations and Electronic Effects
The following table compares 2,6-Dichloro-4-fluoropyridine with key analogs, highlighting structural differences and their implications:
Physicochemical Properties
- Solubility: Fluorine and trifluoromethyl substituents increase hydrophobicity, reducing water solubility compared to amino- or carboxylic acid-containing derivatives.
- Melting Points : Halogenated derivatives generally exhibit higher melting points due to strong intermolecular forces. For example, 3,5-Dichloro-2,6-difluoro-4-pyridinamine (MW 198.99 g/mol) likely has a higher melting point than methyl-substituted analogs .
Biological Activity
2,6-Dichloro-4-fluoropyridine is an organic compound characterized by its unique structure, featuring two chlorine atoms and one fluorine atom attached to a pyridine ring. This compound has garnered attention in medicinal chemistry due to its potential biological activities, particularly in the development of pharmaceuticals and agrochemicals.
The compound exhibits significant biochemical properties that enable it to interact with various enzymes and proteins in biological systems. Its ability to participate in substitution reactions allows it to replace hydrogen atoms in other molecules, facilitating the formation of new compounds. Notably, this compound interacts with cytochrome P450 enzymes, leading to the formation of reactive intermediates that can further react with cellular nucleophiles.
Cellular Effects
Research indicates that this compound influences cellular functions by modulating signaling pathways and gene expression. It has been shown to inhibit specific kinases, resulting in altered phosphorylation states of critical signaling proteins. Such modulation can have downstream effects on cellular metabolism and proliferation.
Molecular Mechanisms
At the molecular level, this compound exerts its biological effects through binding interactions with target biomolecules. This binding can either inhibit or activate enzyme activity depending on the nature of the interaction. The compound's structure plays a crucial role in determining its binding affinity and specificity for various targets.
Temporal and Dosage Effects
The stability and degradation of this compound over time significantly influence its biological activity. In vitro studies show that the compound can undergo hydrolysis and oxidation, leading to degradation products that may possess different biological properties. Furthermore, dosage variations in animal models reveal that lower doses may exhibit minimal toxicity while higher doses can induce adverse effects such as hepatotoxicity and nephrotoxicity.
Metabolic Pathways
The metabolic pathways involving this compound include biotransformation mediated by cytochrome P450 enzymes. These metabolic processes lead to the formation of hydroxylated and dechlorinated metabolites that may have distinct biological activities compared to the parent compound.
Transport and Distribution
Understanding the transport mechanisms of this compound is essential for predicting its biological effects. The compound can traverse cellular membranes through passive diffusion or active transport mechanisms, which are crucial for its distribution within tissues.
Subcellular Localization
The localization of this compound within subcellular compartments impacts its functionality. Targeting signals or post-translational modifications can direct the compound to specific organelles such as the nucleus or mitochondria, influencing its biological activity.
Scientific Research Applications
This compound has diverse applications across various scientific fields:
- Chemistry : It serves as a versatile building block for synthesizing more complex organic molecules.
- Biology : Utilized as a precursor for bioactive molecule synthesis, aiding in studying various biological processes.
- Medicine : Its derivatives have shown potential as antimicrobial, anticancer, and anti-inflammatory agents.
- Industry : In agrochemicals, it is used for synthesizing effective herbicides and insecticides.
Case Studies
Several case studies highlight the biological activity of this compound:
- Anticancer Activity : A study demonstrated that derivatives of this compound exhibited selective cytotoxicity against cancer cell lines while sparing normal cells.
- Antimicrobial Properties : Research indicated that certain derivatives displayed significant antimicrobial activity against both Gram-positive and Gram-negative bacteria.
- Kinase Inhibition : Investigations showed that this compound could effectively inhibit specific kinases involved in cancer progression.
Q & A
Basic Research Questions
Q. What palladium-catalyzed coupling reactions are commonly used to synthesize halogenated pyridine derivatives, and how can reaction conditions be optimized?
- Methodological Answer : Buchwald-Hartwig amination and Ullmann-type couplings are frequently employed for introducing aryl/amino groups to halogenated pyridines. Optimization involves:
- Catalyst-Ligand Systems : Pd(OAc)₂ with Xantphos or XPhos ligands enhances coupling efficiency for chloro/fluoro-substituted pyridines .
- Bases : Strong bases like Cs₂CO₃ or t-BuONa improve yields (e.g., 75% yield in using Cs₂CO₃) .
- Temperature : Reactions often proceed at 80–110°C in toluene or dioxane .
- Key Data :
Substrate | Catalyst | Ligand | Base | Yield | Reference |
---|---|---|---|---|---|
2,4-Dichloro-5-fluoropyrimidine | Pd(OAc)₂ | Xantphos | Cs₂CO₃ | 75% | |
2,6-Dichloro-3-fluoropyridine | Pd(OAc)₂ | Xantphos | t-BuONa | 22% |
Q. Which spectroscopic techniques are critical for characterizing 2,6-Dichloro-4-fluoropyridine analogs, and how are signals interpreted?
- Methodological Answer :
- ¹H/¹³C NMR : Fluorine and chlorine substituents cause distinct splitting patterns. For example, in 3-fluoropyridines, ¹³C signals show coupling constants (e.g., J = 260 Hz for C-F in ) .
- HRMS : Confirms molecular ion peaks (e.g., [M+H]⁺ at m/z 249.0341 in ) .
- Multi-nuclear NMR (e.g., ¹⁹F) is recommended for resolving complex splitting in polyhalogenated systems .
Q. What safety protocols are recommended for handling halogenated pyridines like this compound?
- Methodological Answer :
- Personal Protective Equipment (PPE) : Gloves, goggles, and lab coats are mandatory. Use fume hoods to avoid inhalation .
- Waste Disposal : Segregate halogenated waste and collaborate with certified disposal agencies to prevent environmental contamination .
- Emergency Measures : For skin contact, wash with water immediately; for eye exposure, rinse for 15 minutes and seek medical help .
Advanced Research Questions
Q. How do electron-withdrawing substituents (e.g., -F, -Cl) influence the reactivity of 2,6-dichloropyridine scaffolds in nucleophilic aromatic substitution (SNAr)?
- Methodological Answer :
- Substituent Effects : Fluorine at the 4-position increases ring electron deficiency, enhancing SNAr reactivity at the 2- and 6-positions. Chlorine’s steric bulk may slow kinetics compared to fluorine .
- Kinetic Studies : Monitor reaction progress via HPLC or in situ NMR to compare substitution rates. For example, trifluoromethyl groups () further activate the ring but introduce steric challenges .
Q. How can researchers resolve contradictions in spectroscopic data (e.g., unexpected NMR splitting) for this compound derivatives?
- Methodological Answer :
- Isomer Analysis : Use 2D NMR (COSY, NOESY) to distinguish geometric isomers (e.g., E/Z isomers in ).
- Computational Validation : Compare experimental ¹³C NMR shifts with DFT-calculated values to confirm assignments .
- Crystallography : Single-crystal X-ray diffraction (as in ) provides unambiguous structural confirmation .
Q. What strategies are effective for isolating and characterizing byproducts in the synthesis of this compound analogs?
- Methodological Answer :
- Chromatography : Use preparative HPLC or flash chromatography to separate isomers (e.g., E/Z mixtures in ).
- HRMS/MS : Fragment ions help identify byproducts (e.g., dehalogenated species or dimerization products).
- Kinetic Profiling : Adjust reaction time and temperature to minimize side reactions (e.g., over-coupling in Pd-catalyzed aminations) .
Q. Data Contradiction and Reproducibility
Q. How should researchers address reproducibility challenges in Pd-catalyzed syntheses of halogenated pyridines?
- Methodological Answer :
- Ligand Purity : Impurities in Xantphos/XPhos ligands can drastically alter yields. Recrystallize ligands before use .
- Oxygen Sensitivity : Degas solvents and use inert atmospheres to prevent Pd catalyst deactivation .
- Batch Variability : Replicate reactions with substrates from multiple suppliers to assess consistency .
Properties
IUPAC Name |
2,6-dichloro-4-fluoropyridine | |
---|---|---|
Source | PubChem | |
URL | https://pubchem.ncbi.nlm.nih.gov | |
Description | Data deposited in or computed by PubChem | |
InChI |
InChI=1S/C5H2Cl2FN/c6-4-1-3(8)2-5(7)9-4/h1-2H | |
Source | PubChem | |
URL | https://pubchem.ncbi.nlm.nih.gov | |
Description | Data deposited in or computed by PubChem | |
InChI Key |
CQSPGXVZLJXXPL-UHFFFAOYSA-N | |
Source | PubChem | |
URL | https://pubchem.ncbi.nlm.nih.gov | |
Description | Data deposited in or computed by PubChem | |
Canonical SMILES |
C1=C(C=C(N=C1Cl)Cl)F | |
Source | PubChem | |
URL | https://pubchem.ncbi.nlm.nih.gov | |
Description | Data deposited in or computed by PubChem | |
Molecular Formula |
C5H2Cl2FN | |
Source | PubChem | |
URL | https://pubchem.ncbi.nlm.nih.gov | |
Description | Data deposited in or computed by PubChem | |
Molecular Weight |
165.98 g/mol | |
Source | PubChem | |
URL | https://pubchem.ncbi.nlm.nih.gov | |
Description | Data deposited in or computed by PubChem | |
Retrosynthesis Analysis
AI-Powered Synthesis Planning: Our tool employs the Template_relevance Pistachio, Template_relevance Bkms_metabolic, Template_relevance Pistachio_ringbreaker, Template_relevance Reaxys, Template_relevance Reaxys_biocatalysis model, leveraging a vast database of chemical reactions to predict feasible synthetic routes.
One-Step Synthesis Focus: Specifically designed for one-step synthesis, it provides concise and direct routes for your target compounds, streamlining the synthesis process.
Accurate Predictions: Utilizing the extensive PISTACHIO, BKMS_METABOLIC, PISTACHIO_RINGBREAKER, REAXYS, REAXYS_BIOCATALYSIS database, our tool offers high-accuracy predictions, reflecting the latest in chemical research and data.
Strategy Settings
Precursor scoring | Relevance Heuristic |
---|---|
Min. plausibility | 0.01 |
Model | Template_relevance |
Template Set | Pistachio/Bkms_metabolic/Pistachio_ringbreaker/Reaxys/Reaxys_biocatalysis |
Top-N result to add to graph | 6 |
Feasible Synthetic Routes
Disclaimer and Information on In-Vitro Research Products
Please be aware that all articles and product information presented on BenchChem are intended solely for informational purposes. The products available for purchase on BenchChem are specifically designed for in-vitro studies, which are conducted outside of living organisms. In-vitro studies, derived from the Latin term "in glass," involve experiments performed in controlled laboratory settings using cells or tissues. It is important to note that these products are not categorized as medicines or drugs, and they have not received approval from the FDA for the prevention, treatment, or cure of any medical condition, ailment, or disease. We must emphasize that any form of bodily introduction of these products into humans or animals is strictly prohibited by law. It is essential to adhere to these guidelines to ensure compliance with legal and ethical standards in research and experimentation.