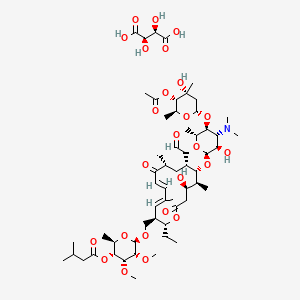
Acetylisovaleryltylosin tartrate
Overview
Description
Tylvalosin, sold under the brand name Aiviosin, is a macrolide antibiotic primarily used in veterinary medicine. It is effective against bacterial infections caused by Mycoplasma hyopneumoniae in swine, which leads to enzootic pneumonia . Tylvalosin is often used in the form of its tartrate salt to enhance its solubility and bioavailability .
Mechanism of Action
Target of Action
Acetylisovaleryltylosin tartrate, also known as tylvalosin, is a macrolide antibiotic . Its primary targets are Mycoplasma, Spirochetes, most gram-positive bacteria, and some gram-negative bacteria , particularly Mycoplasma . It has a strong antibacterial activity against these microorganisms .
Mode of Action
The antimicrobial activity of this compound is centered in the translation of proteins . It combines with the 50S ribosomal subunit of the target bacteria to achieve reversible binding . This interaction prevents peptide chain elongation, thereby exerting a bacteriostatic and bactericidal effect .
Biochemical Pathways
This compound is synthesized by a bioconversion process using Streptomyces thermotolerans with tylosin as the substrate . In this process, tylosin is the precursor, leucine provides isovaleryl, and glucose provides acetyl groups, energy, and maintains the pH of the solution .
Pharmacokinetics
The pharmacokinetics of this compound has been studied in laying hens . After oral administration, the disposition half-life (t 1/2α) of the drug was 0.74±0.071h, whereas the elimination half-life (t 1/2β) of the drug was 2.27±0.41h . The area under the serum concentration-time curve (AUC) was 0.68±0.096μg·h/mL . The total clearance of this compound (CL b) was estimated to be 29.36±2.12L/h/kg . The time-point of maximum plasma concentration of the drug (T p) and the maximum plasma concentration (C max) were calculated as 0.86±0.039h and 0.22±0.052μg/mL . The bioavailability was 60.26±4.72% .
Result of Action
This compound has been found to induce apoptosis . It also has anti-inflammatory activity, relieves oxidative stress, and alleviates acute lung injury by inhibiting NF-κB activation . In addition, it has been observed that this compound significantly reduced S. aureus biofilm formation .
Action Environment
The action of this compound can be influenced by environmental factors. For instance, the bioconversion process of this compound requires a specific pH, which is maintained by glucose . Furthermore, the efficacy of this compound against T. gondii was found to be potent both in vitro and in vivo .
Biochemical Analysis
Biochemical Properties
Acetylisovaleryltylosin tartrate plays a significant role in biochemical reactions by interacting with various enzymes, proteins, and other biomolecules. It exhibits strong antimicrobial activity against Gram-positive bacteria, mycoplasmas, and certain Gram-negative bacteria . The compound binds to the 50S ribosomal subunit of bacterial ribosomes, inhibiting protein synthesis and leading to bacterial cell death . Additionally, this compound has been shown to induce apoptosis in porcine neutrophils by increasing caspase-3 cleavage .
Cellular Effects
This compound influences various cellular processes and functions. It has been observed to induce apoptosis in porcine monocyte-derived macrophages in a concentration- and time-dependent manner . The compound also promotes phagocytosis of porcine neutrophils by macrophages without altering the phagocytic process . Furthermore, this compound exhibits anti-inflammatory activity by inhibiting NF-κB activation, which helps alleviate acute lung injury and reduce oxidative stress .
Molecular Mechanism
The molecular mechanism of this compound involves its binding interactions with bacterial ribosomes. By binding to the 50S ribosomal subunit, the compound inhibits the translocation of peptides during protein synthesis, effectively halting bacterial growth . This mechanism of action is similar to other macrolide antibiotics, but this compound has been chemically modified to enhance its efficacy and reduce resistance . Additionally, the compound’s ability to induce apoptosis and inhibit NF-κB activation contributes to its anti-inflammatory and immunomodulatory effects .
Temporal Effects in Laboratory Settings
In laboratory settings, the effects of this compound have been studied over time to understand its stability, degradation, and long-term impact on cellular function. The compound is rapidly absorbed and distributed throughout the body, with peak concentrations observed in the blood within 30 minutes of administration . Its primary metabolites are distributed in major organs such as the liver, kidneys, and bile . Long-term studies have shown that this compound maintains its antimicrobial activity and continues to exhibit anti-inflammatory effects over extended periods .
Dosage Effects in Animal Models
The effects of this compound vary with different dosages in animal models. At therapeutic doses, the compound effectively treats respiratory infections in swine and poultry without causing significant adverse effects . At higher doses, this compound may exhibit toxic effects, including gastrointestinal disturbances and hepatotoxicity . It is crucial to determine the optimal dosage to maximize therapeutic benefits while minimizing potential risks.
Metabolic Pathways
This compound is involved in various metabolic pathways, primarily related to its antimicrobial activity. The compound is metabolized in the liver, where it undergoes biotransformation to produce active metabolites . These metabolites contribute to the compound’s prolonged antimicrobial effects and are excreted through bile and urine . The interaction of this compound with liver enzymes and cofactors plays a crucial role in its metabolism and overall efficacy.
Transport and Distribution
The transport and distribution of this compound within cells and tissues are facilitated by specific transporters and binding proteins. The compound is rapidly absorbed and distributed to various tissues, with high concentrations observed in the liver, kidneys, and bile . Its localization in these organs is essential for its antimicrobial and anti-inflammatory effects. Additionally, this compound’s ability to cross cellular membranes and reach target sites contributes to its therapeutic efficacy.
Subcellular Localization
This compound exhibits specific subcellular localization, which influences its activity and function. The compound is primarily localized in the cytoplasm and ribosomes of bacterial cells, where it exerts its antimicrobial effects by inhibiting protein synthesis . In mammalian cells, this compound is distributed in the cytoplasm and interacts with various cellular components to induce apoptosis and modulate inflammatory responses . The compound’s subcellular localization is crucial for its overall therapeutic action.
Preparation Methods
Synthetic Routes and Reaction Conditions: Tylvalosin can be synthesized through a bioconversion process using Streptomyces thermotolerans with tylosin as the substrate . The bioconversion process involves the following steps:
Fermentation: Tylosin is fermented using Streptomyces thermotolerans, resulting in a yield of 8148 U/mL.
Recrystallization: The crude tylvalosin is obtained from an acid and alkali recrystallization of the fermentation broth.
Industrial Production Methods: The industrial production of tylvalosin involves large-scale fermentation, followed by purification and recrystallization processes to ensure high yield and purity .
Chemical Reactions Analysis
Types of Reactions: Tylvalosin undergoes various chemical reactions, including:
Oxidation: Tylvalosin can be oxidized under specific conditions to form oxidized derivatives.
Reduction: Reduction reactions can be used to modify the functional groups in tylvalosin.
Substitution: Tylvalosin can undergo substitution reactions, particularly at the hydroxyl and acetyl groups.
Common Reagents and Conditions:
Oxidation: Common oxidizing agents include potassium permanganate and hydrogen peroxide.
Reduction: Reducing agents such as sodium borohydride and lithium aluminum hydride are used.
Substitution: Reagents like acetic anhydride and isovaleryl chloride are used for acetylation and isovaleryl substitution, respectively.
Major Products: The major products formed from these reactions include various derivatives of tylvalosin with modified functional groups, enhancing its antibacterial properties .
Scientific Research Applications
Pharmacological Properties
Mechanism of Action
Acetylisovaleryltylosin tartrate works by inhibiting protein synthesis in bacteria. It binds reversibly to the 50S ribosomal subunit, thus preventing translocation during protein synthesis, which is crucial for bacterial growth and replication. This mechanism makes it effective against rapidly dividing organisms, classifying it as bacteriostatic and mycoplasmastatic .
Pharmacokinetics
The drug is administered orally through medicated feed, with a recommended dosage of 2.125 mg per kg of body weight per day. This dosage is typically incorporated into feed at a concentration of 42.5 mg/kg . It has a withdrawal period of two days for meat and offal, ensuring safety for consumption post-treatment .
Veterinary Applications
Treatment of Swine Enzootic Pneumonia
One of the primary applications of this compound is in the treatment and prevention of Swine Enzootic Pneumonia caused by Mycoplasma hyopneumoniae. Clinical studies have shown that at recommended doses, the drug reduces lung lesions and weight loss in infected pigs, although it does not completely eliminate the infection .
Control of Other Bacterial Infections
Beyond its use in swine pneumonia, this compound has been effective against various bacterial infections in livestock. It has been used to treat infections caused by Mycoplasma gallisepticum and Brachyspira species, showcasing its broad-spectrum antibacterial activity .
Efficacy Against PRRSV Infection
Recent studies have indicated that this compound can enhance the health status of swine herds during Porcine Reproductive and Respiratory Syndrome Virus (PRRSV) vaccination. The compound not only controls bacterial infections but also improves macrophage phagocytic activity and reduces oxidative stress in pigs .
Anti-Toxoplasma Activity
In vitro studies have demonstrated that this compound exhibits potent anti-Toxoplasma activity. It was found to inhibit the growth of Toxoplasma gondii, with an effective concentration (EC50) significantly lower than that of traditional treatments. This suggests potential applications beyond veterinary medicine into human health contexts .
Comparative Data Table
Application | Target Organism | Effectiveness | Administration Route |
---|---|---|---|
Treatment for Swine Enzootic Pneumonia | Mycoplasma hyopneumoniae | Reduces lung lesions and weight loss | Oral (medicated feed) |
Control of Mycoplasma Infections | Mycoplasma gallisepticum | Effective treatment | Oral (medicated feed) |
Anti-Toxoplasma Activity | Toxoplasma gondii | Potent inhibitory effect | In vitro studies |
Enhancement during PRRSV Vaccination | PRRSV | Improves herd health | Oral (medicated feed) |
Comparison with Similar Compounds
Tylosin: Tylvalosin is a derivative of tylosin, with modifications at the hydroxyl and acetyl groups.
Erythromycin: Another macrolide antibiotic with a similar mechanism of action but different spectrum of activity.
Azithromycin: A macrolide with a broader spectrum of activity and longer half-life compared to tylvalosin.
Uniqueness of Tylvalosin: Tylvalosin is unique due to its enhanced activity against Mycoplasma species and its improved pharmacokinetic properties, such as better solubility and bioavailability .
Biological Activity
Acetylisovaleryltylosin tartrate (ATLL) is a semi-synthetic macrolide antibiotic derived from tylosin, primarily utilized in veterinary medicine for the treatment and prevention of bacterial infections in livestock and poultry. This article explores its biological activity, focusing on its antibacterial properties, immunomodulatory effects, and potential applications in treating various infections.
- Molecular Formula : C₄₇H₇₅NO₁₇·C₄H₆O₆
- Appearance : White or yellowish crystalline powder
- Solubility : Slightly soluble in water, ethanol, and methanol; insoluble in chloroform and ether
- Melting Point : 160-165°C
- Density : 1.41 g/cm³
ATLL exerts its antibacterial effects by inhibiting bacterial protein synthesis. It binds to the 50S ribosomal subunit, preventing peptide chain elongation, which is crucial for bacterial growth and replication. This action primarily targets rapidly dividing organisms, making it effective against various pathogens.
Antibacterial Activity
ATLL demonstrates strong antibacterial activity against:
- Gram-positive bacteria : Particularly effective against Mycoplasma species.
- Gram-negative bacteria : Some efficacy noted against Pasteurella and other pathogens.
- Protozoal pathogens : Showed potential as a prophylactic agent against certain protozoal infections.
Table 1: Antibacterial Spectrum of this compound
Pathogen Type | Specific Pathogens | Activity Level |
---|---|---|
Gram-positive | Mycoplasma spp. | High |
Gram-negative | Pasteurella, Escherichia coli | Moderate |
Protozoa | Toxoplasma gondii | High |
Immunomodulatory Effects
Recent studies indicate that ATLL not only acts as an antibiotic but also enhances immune responses in animals. It stimulates the production of phagocytes, particularly macrophages, which play a critical role in the immune defense against infections.
Case Study: Effects on Swine Herds
A study published in December 2022 demonstrated that ATLL administration improved the health status of swine herds affected by Porcine Reproductive and Respiratory Syndrome Virus (PRRSV). Key findings included:
- Dynamic Changes in White Blood Cell Counts : Increased counts were observed post-treatment.
- Cytokine Levels : Elevated serum levels of IFN-γ were noted, indicating enhanced immune activation.
- T Cell Populations : The treatment helped maintain CD8+ T cell levels during vaccination challenges .
Efficacy Against Toxoplasmosis
In vitro and in vivo studies have shown that ATLL exhibits significant anti-Toxoplasma gondii activity. The half-maximal effective concentration (EC50) was determined to be 10.67 μM, indicating potent inhibitory effects on intracellular growth of the parasite. This suggests potential therapeutic applications beyond traditional veterinary uses .
Table 2: Comparative Efficacy of ATLL Against Toxoplasma gondii
Treatment | EC50 (μM) | Effect Type |
---|---|---|
This compound (ATLL) | 10.67 | Intracellular growth inhibition |
Tilmicosin | 17.96 | Extracellular suppression |
Safety Profile and Resistance Considerations
ATLL has a low toxicity profile, making it safe for both animals and humans when used appropriately. However, as with all antibiotics, there is a potential risk for the development of resistance. Current evidence suggests that resistance to ATLL by Mycoplasma hyopneumoniae has not been reported in the field .
Properties
CAS No. |
63428-13-7 |
---|---|
Molecular Formula |
C57H93NO25 |
Molecular Weight |
1192.3 g/mol |
IUPAC Name |
[(2S,3S,4R,6S)-6-[(2R,3S,4R,5R,6R)-6-[[(4R,5S,6S,7R,9R,11E,13E,15R,16R)-4-acetyloxy-16-ethyl-15-[[(2R,3R,4R,5R,6R)-5-hydroxy-3,4-dimethoxy-6-methyloxan-2-yl]oxymethyl]-5,9,13-trimethyl-2,10-dioxo-7-(2-oxoethyl)-1-oxacyclohexadeca-11,13-dien-6-yl]oxy]-4-(dimethylamino)-5-hydroxy-2-methyloxan-3-yl]oxy-4-hydroxy-2,4-dimethyloxan-3-yl] 3-methylbutanoate;(2R,3R)-2,3-dihydroxybutanedioic acid |
InChI |
InChI=1S/C53H87NO19.C4H6O6/c1-16-38-36(26-65-52-49(64-15)48(63-14)44(60)31(7)67-52)22-28(4)17-18-37(57)29(5)23-35(19-20-55)46(30(6)39(69-34(10)56)24-41(59)70-38)73-51-45(61)43(54(12)13)47(32(8)68-51)72-42-25-53(11,62)50(33(9)66-42)71-40(58)21-27(2)3;5-1(3(7)8)2(6)4(9)10/h17-18,20,22,27,29-33,35-36,38-39,42-52,60-62H,16,19,21,23-26H2,1-15H3;1-2,5-6H,(H,7,8)(H,9,10)/b18-17+,28-22+;/t29-,30+,31-,32-,33+,35+,36-,38-,39-,42+,43-,44-,45-,46-,47-,48-,49-,50+,51+,52-,53-;1-,2-/m11/s1 |
InChI Key |
OLLSDNUHBJHKJS-XKORHJEPSA-N |
SMILES |
CCC1C(C=C(C=CC(=O)C(CC(C(C(C(CC(=O)O1)O)C)OC2C(C(C(C(O2)C)OC3CC(C(C(O3)C)OC(=O)C)(C)O)N(C)C)O)CC=O)C)C)COC4C(C(C(C(O4)C)OC(=O)CC(C)C)OC)OC.C(C(C(=O)O)O)(C(=O)O)O |
Isomeric SMILES |
CC[C@@H]1[C@H](/C=C(/C=C/C(=O)[C@@H](C[C@@H]([C@@H]([C@H]([C@@H](CC(=O)O1)OC(=O)C)C)O[C@H]2[C@@H]([C@H]([C@@H]([C@H](O2)C)O[C@H]3C[C@@]([C@H]([C@@H](O3)C)OC(=O)CC(C)C)(C)O)N(C)C)O)CC=O)C)\C)CO[C@H]4[C@@H]([C@@H]([C@@H]([C@H](O4)C)O)OC)OC.[C@@H]([C@H](C(=O)O)O)(C(=O)O)O |
Canonical SMILES |
CCC1C(C=C(C=CC(=O)C(CC(C(C(C(CC(=O)O1)OC(=O)C)C)OC2C(C(C(C(O2)C)OC3CC(C(C(O3)C)OC(=O)CC(C)C)(C)O)N(C)C)O)CC=O)C)C)COC4C(C(C(C(O4)C)O)OC)OC.C(C(C(=O)O)O)(C(=O)O)O |
Origin of Product |
United States |
Retrosynthesis Analysis
AI-Powered Synthesis Planning: Our tool employs the Template_relevance Pistachio, Template_relevance Bkms_metabolic, Template_relevance Pistachio_ringbreaker, Template_relevance Reaxys, Template_relevance Reaxys_biocatalysis model, leveraging a vast database of chemical reactions to predict feasible synthetic routes.
One-Step Synthesis Focus: Specifically designed for one-step synthesis, it provides concise and direct routes for your target compounds, streamlining the synthesis process.
Accurate Predictions: Utilizing the extensive PISTACHIO, BKMS_METABOLIC, PISTACHIO_RINGBREAKER, REAXYS, REAXYS_BIOCATALYSIS database, our tool offers high-accuracy predictions, reflecting the latest in chemical research and data.
Strategy Settings
Precursor scoring | Relevance Heuristic |
---|---|
Min. plausibility | 0.01 |
Model | Template_relevance |
Template Set | Pistachio/Bkms_metabolic/Pistachio_ringbreaker/Reaxys/Reaxys_biocatalysis |
Top-N result to add to graph | 6 |
Feasible Synthetic Routes
Q1: What is the mechanism of action of Acetylisovaleryltylosin Tartrate (ATLL) as an antibacterial agent?
A1: ATLL is a macrolide antibiotic that inhibits bacterial protein synthesis. It binds to the 50S ribosomal subunit of susceptible bacteria, blocking the translocation step of protein synthesis. [] This halts bacterial growth and can lead to bacterial cell death.
Q2: How effective is ATLL against biofilm formation compared to other antibiotics?
A2: Research suggests that ATLL, unlike other tested antibiotics like kanamycin and enrofloxacin, does not effectively inhibit biofilm formation in Staphylococcus aureus. In fact, ATLL was shown to upregulate the expression of biofilm-related genes (sarA, fnbA, rbf, lrgA, cidA, and eno) in S. aureus, potentially promoting biofilm formation. []
Q3: Are there alternative purification methods for ATLL besides crystallization?
A3: Yes, silica gel chromatography has been investigated as a purification method for ATLL. This method uses a mixture of petroleum ether, ethyl acetate/butyl acetate, and amines (diethylamine, triethylamine, or N,N-diisopropylethylamine) as the eluent. This technique has been shown to effectively remove impurities and achieve a high purity of ATLL (>97%). []
Q4: What is the impact of metal ions like Zn2+ and Cu2+ on the interaction between ATLL and Bovine Serum Albumin (BSA)?
A4: Studies using spectroscopic analysis have shown that both Zn2+ and Cu2+ can influence the binding of ATLL to BSA. Zn2+ was found to decrease the binding affinity, possibly due to competition for binding sites on BSA. Conversely, Cu2+ increased ATLL's binding affinity to BSA, potentially through the formation of a Cu2+-ATLL complex. []
Q5: Does ATLL show promising activity against parasites like Toxoplasma gondii?
A5: Yes, in vitro and in vivo studies have demonstrated ATLL's effectiveness against Toxoplasma gondii. ATLL exhibited potent inhibition of intracellular parasite growth, suppressing tachyzoite proliferation and potentially disrupting parasite morphology. Furthermore, ATLL significantly improved survival rates in mice with acute toxoplasmosis. []
Q6: What are some innovative methods being explored for recovering ATLL from crystallization mother liquor?
A6: One method involves adding a surfactant to the crystallization mother liquor and then concentrating it using air bubbles. The collected air bubble films are cooled, and a separating agent is added. This process allows for the separation, filtration, and crystallization of ATLL, leading to the recovery of pure ATLL. [] This method addresses the challenges of low ATLL concentration and its poor thermal stability in the mother liquor.
Disclaimer and Information on In-Vitro Research Products
Please be aware that all articles and product information presented on BenchChem are intended solely for informational purposes. The products available for purchase on BenchChem are specifically designed for in-vitro studies, which are conducted outside of living organisms. In-vitro studies, derived from the Latin term "in glass," involve experiments performed in controlled laboratory settings using cells or tissues. It is important to note that these products are not categorized as medicines or drugs, and they have not received approval from the FDA for the prevention, treatment, or cure of any medical condition, ailment, or disease. We must emphasize that any form of bodily introduction of these products into humans or animals is strictly prohibited by law. It is essential to adhere to these guidelines to ensure compliance with legal and ethical standards in research and experimentation.