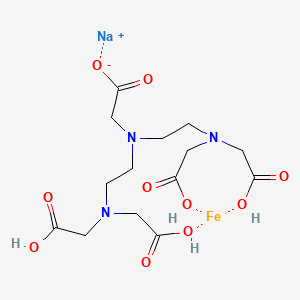
Sodium hydrogen ferric dtpa
- Click on QUICK INQUIRY to receive a quote from our team of experts.
- With the quality product at a COMPETITIVE price, you can focus more on your research.
Overview
Description
Sodium hydrogen ferric diethylenetriaminepentaacetate is a complex compound with the chemical formula C14H19FeN3NaO10. It is known for its chelating properties, which allow it to form stable complexes with metal ions. This compound is commonly used in medical imaging and as a treatment for heavy metal poisoning .
Biochemical Analysis
Biochemical Properties
Sodium hydrogen ferric DTPA plays a crucial role in biochemical reactions by chelating metal ions, thereby preventing metal-catalyzed oxidative damage. It interacts with various enzymes, proteins, and other biomolecules. For instance, it binds to iron ions, forming a stable complex that can be utilized in various biochemical assays and treatments. The nature of these interactions is primarily based on the strong affinity of DTPA for metal ions, which allows it to sequester metals and prevent their participation in harmful reactions .
Cellular Effects
This compound influences various cellular processes by modulating the availability of metal ions. It affects cell signaling pathways, gene expression, and cellular metabolism. By chelating iron, it can reduce the availability of free iron, which is essential for the proliferation of certain cell types. This reduction in free iron can lead to decreased cellular metabolism and altered gene expression, impacting cell function .
Molecular Mechanism
The molecular mechanism of this compound involves its ability to form stable complexes with metal ions. This chelation process inhibits the catalytic activity of metal-dependent enzymes and prevents metal-induced oxidative stress. This compound binds to iron ions through its carboxylate and amine groups, forming a hexadentate complex that is highly stable. This binding interaction can inhibit enzymes that require metal ions as cofactors, thereby modulating biochemical pathways .
Temporal Effects in Laboratory Settings
In laboratory settings, the effects of this compound can change over time due to its stability and degradation. The compound is relatively stable under standard laboratory conditions, but its efficacy can diminish over extended periods. Long-term studies have shown that this compound can maintain its chelating activity for several weeks, but gradual degradation can lead to reduced effectiveness. This degradation can impact cellular functions, as the availability of free metal ions may increase over time .
Dosage Effects in Animal Models
The effects of this compound vary with different dosages in animal models. At low doses, it effectively chelates metal ions without causing significant toxicity. At high doses, it can lead to adverse effects such as renal toxicity and disruption of essential metal ion homeostasis. Studies have shown that there is a threshold dose beyond which the toxic effects outweigh the benefits, highlighting the importance of dose optimization in therapeutic applications .
Metabolic Pathways
This compound is involved in various metabolic pathways, primarily through its interaction with metal ions. It can influence metabolic flux by altering the availability of metal cofactors required for enzymatic reactions. For example, by chelating iron, it can affect the activity of iron-dependent enzymes involved in cellular respiration and DNA synthesis. This modulation of metabolic pathways can lead to changes in metabolite levels and overall cellular metabolism .
Transport and Distribution
Within cells and tissues, this compound is transported and distributed through various mechanisms. It can interact with transporters and binding proteins that facilitate its movement across cellular membranes. Once inside the cell, it can localize to specific compartments where it exerts its chelating activity. The distribution of this compound is influenced by its affinity for metal ions and the presence of specific transporters that recognize the chelated complex .
Subcellular Localization
The subcellular localization of this compound is critical for its activity and function. It can be directed to specific organelles through targeting signals and post-translational modifications. For instance, it may localize to the mitochondria, where it can chelate iron and prevent oxidative damage. The precise localization within subcellular compartments ensures that this compound exerts its effects in a targeted manner, enhancing its efficacy in biochemical applications .
Preparation Methods
Synthetic Routes and Reaction Conditions: Sodium hydrogen ferric diethylenetriaminepentaacetate is synthesized by reacting diethylenetriaminepentaacetic acid with ferric chloride and sodium hydroxide. The reaction typically occurs in an aqueous medium under controlled pH conditions to ensure the formation of the desired complex .
Industrial Production Methods: In industrial settings, the production of sodium hydrogen ferric diethylenetriaminepentaacetate involves large-scale reactions in reactors equipped with pH control systems. The reactants are mixed in precise stoichiometric ratios, and the reaction mixture is stirred continuously to ensure complete complexation. The product is then purified through filtration and crystallization processes .
Chemical Reactions Analysis
Types of Reactions: Sodium hydrogen ferric diethylenetriaminepentaacetate primarily undergoes chelation reactions, where it forms stable complexes with various metal ions. It can also participate in substitution reactions where the metal ion in the complex is replaced by another metal ion .
Common Reagents and Conditions:
Chelation Reactions: Typically involve metal salts such as ferric chloride, cupric sulfate, or zinc acetate in aqueous solutions.
Substitution Reactions: Involve the use of competing metal ions under controlled pH conditions to facilitate the exchange of metal ions in the complex.
Major Products Formed: The major products formed from these reactions are the metal complexes of sodium hydrogen ferric diethylenetriaminepentaacetate, such as ferric, cupric, and zinc complexes .
Scientific Research Applications
Sodium hydrogen ferric diethylenetriaminepentaacetate has a wide range of applications in scientific research:
Mechanism of Action
The mechanism by which sodium hydrogen ferric diethylenetriaminepentaacetate exerts its effects involves the formation of stable complexes with metal ions. The compound has multiple coordination sites that bind to metal ions, forming highly stable chelates. This chelation process prevents the metal ions from participating in unwanted chemical reactions and facilitates their removal from the system through excretion .
Comparison with Similar Compounds
Ethylenediaminetetraacetic acid (EDTA): Another widely used chelating agent with similar properties but fewer coordination sites compared to diethylenetriaminepentaacetic acid.
Nitrilotriacetic acid (NTA): A chelating agent with fewer coordination sites and lower stability constants for metal complexes compared to diethylenetriaminepentaacetic acid.
Uniqueness: Sodium hydrogen ferric diethylenetriaminepentaacetate is unique due to its high affinity for metal ions and its ability to form highly stable complexes. This makes it particularly effective in applications requiring strong and stable chelation, such as medical imaging and heavy metal detoxification .
Properties
CAS No. |
12389-75-2 |
---|---|
Molecular Formula |
C14H19FeN3NaO10+3 |
Molecular Weight |
468.15 g/mol |
IUPAC Name |
sodium;2-[bis[2-[bis(carboxylatomethyl)amino]ethyl]amino]acetate;hydron;iron(6+) |
InChI |
InChI=1S/C14H23N3O10.Fe.Na/c18-10(19)5-15(1-3-16(6-11(20)21)7-12(22)23)2-4-17(8-13(24)25)9-14(26)27;;/h1-9H2,(H,18,19)(H,20,21)(H,22,23)(H,24,25)(H,26,27);;/q;+6;+1/p-4 |
InChI Key |
UDEONADEAJJCCV-UHFFFAOYSA-J |
SMILES |
C(CN(CC(=O)O)CC(=O)O)N(CCN(CC(=O)O)CC(=O)O)CC(=O)[O-].[Na+].[Fe] |
Canonical SMILES |
[H+].C(CN(CC(=O)[O-])CC(=O)[O-])N(CCN(CC(=O)[O-])CC(=O)[O-])CC(=O)[O-].[Na+].[Fe+6] |
Key on ui other cas no. |
12389-75-2 |
physical_description |
Dry Powder, Liquid; Dry Powder; Other Solid; NKRA; Pellets or Large Crystals |
Origin of Product |
United States |
Retrosynthesis Analysis
AI-Powered Synthesis Planning: Our tool employs the Template_relevance Pistachio, Template_relevance Bkms_metabolic, Template_relevance Pistachio_ringbreaker, Template_relevance Reaxys, Template_relevance Reaxys_biocatalysis model, leveraging a vast database of chemical reactions to predict feasible synthetic routes.
One-Step Synthesis Focus: Specifically designed for one-step synthesis, it provides concise and direct routes for your target compounds, streamlining the synthesis process.
Accurate Predictions: Utilizing the extensive PISTACHIO, BKMS_METABOLIC, PISTACHIO_RINGBREAKER, REAXYS, REAXYS_BIOCATALYSIS database, our tool offers high-accuracy predictions, reflecting the latest in chemical research and data.
Strategy Settings
Precursor scoring | Relevance Heuristic |
---|---|
Min. plausibility | 0.01 |
Model | Template_relevance |
Template Set | Pistachio/Bkms_metabolic/Pistachio_ringbreaker/Reaxys/Reaxys_biocatalysis |
Top-N result to add to graph | 6 |
Feasible Synthetic Routes
Disclaimer and Information on In-Vitro Research Products
Please be aware that all articles and product information presented on BenchChem are intended solely for informational purposes. The products available for purchase on BenchChem are specifically designed for in-vitro studies, which are conducted outside of living organisms. In-vitro studies, derived from the Latin term "in glass," involve experiments performed in controlled laboratory settings using cells or tissues. It is important to note that these products are not categorized as medicines or drugs, and they have not received approval from the FDA for the prevention, treatment, or cure of any medical condition, ailment, or disease. We must emphasize that any form of bodily introduction of these products into humans or animals is strictly prohibited by law. It is essential to adhere to these guidelines to ensure compliance with legal and ethical standards in research and experimentation.