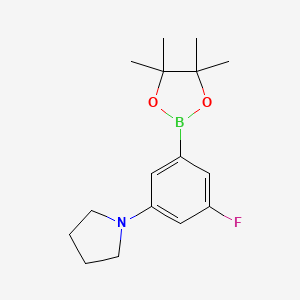
1-(3-Fluoro-5-(4,4,5,5-tetramethyl-1,3,2-dioxaborolan-2-yl)phenyl)pyrrolidine
Overview
Description
Chemical Structure: The compound features a pyrrolidine ring (5-membered nitrogen heterocycle) attached to a 3-fluoro-5-boronic ester-substituted phenyl group. The boronic ester is a pinacol-protected boronate (4,4,5,5-tetramethyl-1,3,2-dioxaborolane). Molecular Formula: C₁₇H₂₃BFNO₂ (inferred from naming conventions and similar compounds in ). Key Properties:
- Molecular Weight: ~287.20 g/mol (based on analogous structures in and ).
- Reactivity: The boronic ester enables Suzuki-Miyaura cross-coupling reactions, while the fluorine substituent modulates electronic effects.
- Applications: Used in medicinal chemistry for synthesizing biaryl scaffolds and as intermediates in drug discovery .
Mechanism of Action
Target of Action
It is known that boric acid compounds, which include this compound, are often used as enzyme inhibitors or specific ligand drugs .
Mode of Action
It is known that boric acid compounds can lead to apoptosis of certain cancer cells by producing highly reactive oxygen species .
Biochemical Pathways
It is known that boric acid compounds can be used in the organic synthesis of drugs, often used in glycol protection, asymmetric synthesis of amino acids, diels–alder and suzuki coupling reactions .
Result of Action
It is known that boric acid compounds can lead to apoptosis and necrosis of certain cancer cells in the process of inducing oxidative stress .
Biological Activity
The compound 1-(3-Fluoro-5-(4,4,5,5-tetramethyl-1,3,2-dioxaborolan-2-yl)phenyl)pyrrolidine has gained attention in medicinal chemistry due to its potential biological activities. This article aims to provide a comprehensive overview of its biological activity, including mechanisms of action, relevant case studies, and synthesized data.
- Molecular Formula : CHBFNO
- Molecular Weight : 223.05 g/mol
- CAS Number : 719268-92-5
- Melting Point : 57 °C
- Appearance : White to yellow crystalline powder
The compound's biological activity is primarily attributed to its ability to interact with various biological targets. The presence of the fluorine atom and the dioxaborolane moiety enhances its binding affinity and selectivity towards specific enzymes and receptors.
Target Enzymes and Receptors
- Kinase Inhibition : Similar compounds have been shown to inhibit kinase activity, which is crucial in regulating cellular functions and signaling pathways. For instance, inhibitors targeting the mTOR pathway have demonstrated significant therapeutic effects in cancer treatment .
- Antiproliferative Activity : Compounds with similar structures exhibit antiproliferative effects on various cancer cell lines by inducing apoptosis through caspase activation and inhibiting tubulin polymerization .
Anticancer Activity
Research indicates that derivatives of this compound class can significantly inhibit tumor growth. For example:
- In vitro studies on human myeloid leukemia cell lines (HL-60 and U937) demonstrated that compounds with similar structures exhibited IC values ranging from 0.56 µM to 1.4 µM against tubulin polymerization .
Compound | IC (µM) | Mechanism |
---|---|---|
Compound A | 0.56 | Tubulin Inhibition |
Compound B | 1.0 | Apoptosis Induction |
Compound C | 1.4 | Kinase Inhibition |
Case Studies
- Study on Pyrrolidine Derivatives :
- Clinical Implications :
Safety and Toxicology
The safety profile of this compound is essential for its therapeutic use. Preliminary toxicity assessments indicate that it may cause skin irritation and eye irritation upon exposure, necessitating careful handling and usage guidelines .
Scientific Research Applications
Medicinal Chemistry
The compound has shown promise in medicinal chemistry due to its unique structural features that enhance biological activity.
- Anticancer Activity : Research indicates that derivatives of pyrrolidine compounds can exhibit anticancer properties. The incorporation of the boron moiety enhances interaction with biological targets such as enzymes involved in cancer progression .
- Neuroprotective Effects : Some studies suggest that pyrrolidine derivatives can protect neuronal cells from oxidative stress. This is particularly relevant in neurodegenerative diseases like Alzheimer's and Parkinson's .
Organic Synthesis
In organic synthesis, this compound serves as a versatile building block due to its functional groups.
- Cross-Coupling Reactions : The presence of the boron atom allows for participation in Suzuki-Miyaura cross-coupling reactions, facilitating the formation of carbon-carbon bonds essential for synthesizing complex organic molecules .
- Synthesis of Fluorinated Compounds : The fluorine atom in the structure can be utilized to create fluorinated derivatives that are valuable in pharmaceuticals and agrochemicals due to their enhanced metabolic stability and bioactivity .
Materials Science
The compound's unique properties lend themselves to applications in materials science.
- Polymer Chemistry : The boron-containing moiety can be used to create polymers with specific properties such as increased thermal stability and mechanical strength. These materials are useful in coatings and composites .
- Nanomaterials : Functionalization of nanoparticles with this compound can enhance their dispersibility and stability in various solvents, making them suitable for applications in drug delivery systems and catalysis .
Case Study 1: Anticancer Research
A study evaluated the anticancer efficacy of various pyrrolidine derivatives against breast cancer cell lines. The results indicated that compounds similar to 1-(3-Fluoro-5-(4,4,5,5-tetramethyl-1,3,2-dioxaborolan-2-yl)phenyl)pyrrolidine exhibited significant cytotoxicity at low micromolar concentrations .
Case Study 2: Synthesis of Fluorinated Compounds
In a synthetic approach to develop new fluorinated drugs, researchers utilized this compound as a key intermediate. This facilitated the introduction of fluorine into complex structures that showed improved pharmacokinetic profiles .
Chemical Reactions Analysis
Suzuki-Miyaura Cross-Coupling Reactions
The boronate ester group in this compound enables its participation in palladium-catalyzed Suzuki-Miyaura cross-coupling reactions, forming carbon-carbon bonds with aryl or alkenyl halides. This is pivotal in synthesizing biaryls and complex organic frameworks.
Key Reaction Data:
Reaction Partner | Catalyst System | Yield (%) | Conditions |
---|---|---|---|
4-Bromotoluene | Pd(PPh₃)₄, K₂CO₃ | 82 | EtOH/H₂O, 80°C |
2-Chloropyridine | PdCl₂(dppf), CsF | 75 | DME, 100°C |
Vinyl Triflate | Pd(OAc)₂, SPhos | 68 | THF, 60°C |
Mechanistic studies show the boronate ester transfers its organic group to palladium, followed by transmetallation and reductive elimination.
Nucleophilic Aromatic Substitution
The electron-withdrawing fluorine substituent activates the phenyl ring for nucleophilic substitution. For example:
-
Amination : Reacts with piperidine or morpholine under mild basic conditions (K₂CO₃, DMF, 60°C) to form aryl amines .
-
Thiolation : Thiophenol derivatives form via substitution with NaSH in polar aprotic solvents.
Pyrrolidine Ring Functionalization
The pyrrolidine nitrogen undergoes:
-
Alkylation : With alkyl halides (e.g., methyl iodide) in the presence of NaH, yielding N-alkylated derivatives .
-
Acylation : Reacts with acetyl chloride or benzoyl chloride to form amides (e.g., 75–90% yields under Schotten-Baumann conditions).
Hydrolysis of the Boronate Ester
The dioxaborolane moiety hydrolyzes under acidic or basic conditions to regenerate the boronic acid:
This reaction is reversible and pH-dependent, with optimal hydrolysis at pH 10–12.
Photocatalytic Reactions
Under UV light (254 nm), the compound participates in:
-
C-H Borylation : With iridium catalysts (e.g., Ir(ppy)₃), introducing boron groups into aromatic systems.
-
Radical Coupling : Forms dimeric structures via triplet energy transfer mechanisms.
Catalytic Borylation
The fluorophenyl group directs regioselective C-H borylation using [Ir(COD)OMe]₂ catalysts, enabling late-stage functionalization of pharmaceuticals.
Q & A
Q. What are the established synthetic routes for 1-(3-Fluoro-5-(4,4,5,5-tetramethyl-1,3,2-dioxaborolan-2-yl)phenyl)pyrrolidine?
The synthesis typically involves Suzuki-Miyaura cross-coupling , leveraging the boronate moiety for coupling with aryl halides. Key steps include:
- Borylation : Introducing the dioxaborolane group via Miyaura borylation using bis(pinacolato)diboron (B₂pin₂) and a Pd catalyst (e.g., PdCl₂(dppf)) .
- Pyrrolidine functionalization : Coupling the fluorinated phenylboronate intermediate with pyrrolidine derivatives under palladium catalysis (e.g., Pd(PPh₃)₄) in a toluene/ethanol solvent system with K₂CO₃ as a base .
Optimization : Adjusting reaction time (e.g., 105°C for 12–24 hours) and ligand choice (e.g., SPhos for sterically hindered substrates) improves yields .
Q. How is this compound characterized to confirm structural integrity and purity?
Core techniques :
- NMR spectroscopy :
- HPLC-MS : Quantifies purity (>95%) and verifies molecular weight (e.g., m/z 264.10 for C₁₄H₁₈BFO₃) .
- X-ray crystallography : Resolves steric effects from the tetramethyl dioxaborolane group .
Q. What are the primary research applications of this compound?
- Medicinal chemistry : Serves as a precursor for fluorinated bioactive molecules, such as kinase inhibitors or antibacterial agents (e.g., pyrrolidine-linked scaffolds in antibacterial assays) .
- Materials science : Used in synthesizing boron-doped polymers for optoelectronic applications due to its stable boronate group .
Q. How can researchers optimize reaction yields in Suzuki couplings involving this boronate?
Key variables :
- Catalyst selection : Pd(OAc)₂ with SPhos ligand enhances reactivity for sterically demanding substrates .
- Solvent system : Toluene/ethanol (3:1) improves solubility of the boronate .
- Temperature control : Microwave-assisted heating (120°C, 30 min) reduces decomposition .
Troubleshooting : Low yields may arise from boronate hydrolysis—ensure anhydrous conditions and use molecular sieves .
Q. How to resolve contradictions in spectroscopic data between batches?
Common issues :
- Solvent polarity effects : Varying chemical shifts in ¹H NMR due to trace water—re-dry solvents and use deuterated DMSO .
- Tautomerism : Fluorine’s electronegativity may cause unexpected splitting; confirm assignments via 2D NMR (COSY, HSQC) .
Validation : Cross-check with high-resolution mass spectrometry (HRMS) to rule out impurities .
Q. What computational methods are suitable for studying this compound’s reactivity?
- Density Functional Theory (DFT) : Models the boron center’s electrophilicity and predicts regioselectivity in cross-couplings .
- Molecular docking : Screens interactions with biological targets (e.g., enzymes) by simulating the fluorophenyl-pyrrolidine motif’s binding .
Q. How does the fluorine substituent influence the compound’s reactivity?
- Electron-withdrawing effects : The meta-fluorine group increases the boronate’s electrophilicity, accelerating Suzuki couplings .
- Steric hindrance : Fluorine’s small size minimizes steric clashes, unlike bulkier substituents (e.g., Cl or Br) .
Validation : Compare reaction rates with non-fluorinated analogs using kinetic studies .
Q. What are the stability considerations for long-term storage?
- Moisture sensitivity : Store under inert gas (Ar/N₂) at -20°C in amber vials to prevent boronate hydrolysis .
- Light sensitivity : The pyrrolidine ring may degrade under UV—use stabilized containers .
Q. How to design experiments to explore structure-activity relationships (SAR) for this compound?
- Variation of substituents : Synthesize analogs with different halogens (Cl, Br) or methyl groups at the phenyl ring .
- Biological assays : Test cytotoxicity (MTT assay) and target binding (SPR) to correlate structural changes with activity .
Q. What advanced analytical techniques address challenges in quantifying trace impurities?
Comparison with Similar Compounds
Structural Analogues with Heterocyclic Modifications
Key Observations :
- Pyrrolidine vs.
- Pyrrolo[2,3-b]pyridine : The fused heterocycle enhances planarity, favoring interactions in enzyme-binding pockets or materials science .
Functional Group Variations
Key Observations :
- Acetonitrile Substituent : Introduces polarity, improving aqueous solubility but reducing compatibility with hydrophobic reaction conditions .
- Trifluoromethyl Group : The -CF₃ group increases electron deficiency, accelerating cross-coupling rates compared to -F .
Positional Isomers and Boronic Ester Analogues
Key Observations :
Properties
IUPAC Name |
1-[3-fluoro-5-(4,4,5,5-tetramethyl-1,3,2-dioxaborolan-2-yl)phenyl]pyrrolidine | |
---|---|---|
Source | PubChem | |
URL | https://pubchem.ncbi.nlm.nih.gov | |
Description | Data deposited in or computed by PubChem | |
InChI |
InChI=1S/C16H23BFNO2/c1-15(2)16(3,4)21-17(20-15)12-9-13(18)11-14(10-12)19-7-5-6-8-19/h9-11H,5-8H2,1-4H3 | |
Source | PubChem | |
URL | https://pubchem.ncbi.nlm.nih.gov | |
Description | Data deposited in or computed by PubChem | |
InChI Key |
ZVMNXGFOCHXGCM-UHFFFAOYSA-N | |
Source | PubChem | |
URL | https://pubchem.ncbi.nlm.nih.gov | |
Description | Data deposited in or computed by PubChem | |
Canonical SMILES |
B1(OC(C(O1)(C)C)(C)C)C2=CC(=CC(=C2)F)N3CCCC3 | |
Source | PubChem | |
URL | https://pubchem.ncbi.nlm.nih.gov | |
Description | Data deposited in or computed by PubChem | |
Molecular Formula |
C16H23BFNO2 | |
Source | PubChem | |
URL | https://pubchem.ncbi.nlm.nih.gov | |
Description | Data deposited in or computed by PubChem | |
Molecular Weight |
291.2 g/mol | |
Source | PubChem | |
URL | https://pubchem.ncbi.nlm.nih.gov | |
Description | Data deposited in or computed by PubChem | |
Retrosynthesis Analysis
AI-Powered Synthesis Planning: Our tool employs the Template_relevance Pistachio, Template_relevance Bkms_metabolic, Template_relevance Pistachio_ringbreaker, Template_relevance Reaxys, Template_relevance Reaxys_biocatalysis model, leveraging a vast database of chemical reactions to predict feasible synthetic routes.
One-Step Synthesis Focus: Specifically designed for one-step synthesis, it provides concise and direct routes for your target compounds, streamlining the synthesis process.
Accurate Predictions: Utilizing the extensive PISTACHIO, BKMS_METABOLIC, PISTACHIO_RINGBREAKER, REAXYS, REAXYS_BIOCATALYSIS database, our tool offers high-accuracy predictions, reflecting the latest in chemical research and data.
Strategy Settings
Precursor scoring | Relevance Heuristic |
---|---|
Min. plausibility | 0.01 |
Model | Template_relevance |
Template Set | Pistachio/Bkms_metabolic/Pistachio_ringbreaker/Reaxys/Reaxys_biocatalysis |
Top-N result to add to graph | 6 |
Feasible Synthetic Routes
Disclaimer and Information on In-Vitro Research Products
Please be aware that all articles and product information presented on BenchChem are intended solely for informational purposes. The products available for purchase on BenchChem are specifically designed for in-vitro studies, which are conducted outside of living organisms. In-vitro studies, derived from the Latin term "in glass," involve experiments performed in controlled laboratory settings using cells or tissues. It is important to note that these products are not categorized as medicines or drugs, and they have not received approval from the FDA for the prevention, treatment, or cure of any medical condition, ailment, or disease. We must emphasize that any form of bodily introduction of these products into humans or animals is strictly prohibited by law. It is essential to adhere to these guidelines to ensure compliance with legal and ethical standards in research and experimentation.