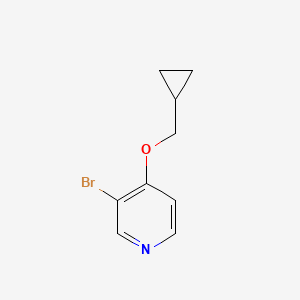
3-Bromo-4-(cyclopropylmethoxy)pyridine
Overview
Description
3-Bromo-4-(cyclopropylmethoxy)pyridine (CAS 1357095-18-1) is a brominated pyridine derivative with the molecular formula C₉H₁₀BrNO and a molecular weight of 228.09 g/mol . It features a cyclopropylmethoxy substituent at the 4-position and a bromine atom at the 3-position of the pyridine ring. This compound is typically stored at 2–8°C under dry conditions to ensure stability . Its primary applications include use as a pharmaceutical intermediate and in organic synthesis, particularly in coupling reactions (e.g., Suzuki-Miyaura) due to the reactivity of the bromine atom .
Preparation Methods
General Synthetic Strategy
The synthesis of 3-bromo-4-(cyclopropylmethoxy)pyridine typically involves two main steps:
- Introduction of the bromine substituent at the 3-position of the pyridine ring.
- Attachment of the cyclopropylmethoxy group at the 4-position via nucleophilic substitution or etherification.
This approach is consistent with general principles of pyridine functionalization, where selective halogenation and alkoxylation are key transformations.
Preparation Methods
Synthesis of 3-Bromo-4-hydroxypyridine or 3-Bromo-4-methoxypyridine as Precursors
Since direct substitution on pyridine can be challenging due to its electron-deficient nature, a common approach is to start from a suitably substituted pyridine intermediate:
Halogenation: Bromination at the 3-position can be achieved by electrophilic aromatic substitution or via diazonium salt intermediates. For example, 4-methyl-3-bromopyridine can be prepared by bromination of 4-methylpyridine derivatives using bromine under controlled conditions.
Methoxylation or Hydroxylation at the 4-position: Introduction of a methoxy group at the 4-position can be done by nucleophilic substitution of a suitable leaving group (e.g., halogen or hydroxyl group) or by methylation of 4-hydroxypyridine derivatives.
Etherification to Introduce the Cyclopropylmethoxy Group
The critical step to obtain this compound is the formation of the ether bond between the 4-position hydroxyl group of a 3-bromopyridine derivative and cyclopropylmethanol or its derivative.
-
- Use of a base (e.g., potassium carbonate or sodium hydride) to deprotonate the hydroxyl group.
- Reaction with cyclopropylmethyl halide (e.g., bromide or chloride) as the alkylating agent.
- Solvent such as dimethylformamide (DMF) or acetonitrile under reflux or elevated temperatures to facilitate nucleophilic substitution.
Alternative methods: Mitsunobu reaction conditions can also be employed for ether formation, especially when sensitive functional groups are present.
Representative Synthetic Route
Step | Reaction Type | Reagents/Conditions | Outcome |
---|---|---|---|
1 | Bromination | Pyridine derivative + Br2, controlled temp | 3-Bromo-substituted pyridine intermediate |
2 | Hydroxylation or Methoxylation | Nucleophilic substitution or methylation | 3-Bromo-4-hydroxypyridine or 3-bromo-4-methoxypyridine |
3 | Etherification | Base (K2CO3), cyclopropylmethyl bromide, DMF, reflux | This compound |
Detailed Research Findings
Catalytic bromination: Using mild conditions such as bromine addition at low temperatures to avoid polybromination and ensure regioselectivity at the 3-position.
Hydrogenation and reduction steps: For related pyridine derivatives, catalytic hydrogenation (e.g., Pd/C or Raney Ni) under mild pressure and temperature conditions can be used to reduce nitro groups to amines, which can then be converted to bromides via diazotization and Sandmeyer reactions.
Ether bond formation: The alkylation of phenolic or pyridinol hydroxyl groups with cyclopropylmethyl halides is well-documented, with yields typically ranging from 70% to 90%, depending on reaction conditions and purification methods.
Purification: Standard purification techniques include extraction, drying with anhydrous agents, and chromatographic methods to isolate the pure compound.
Comparative Analysis with Related Compounds
Summary Table of Preparation Conditions
Industrial and Research Considerations
The described methods allow for scalable synthesis suitable for industrial production due to mild reaction conditions and high yields.
The choice of catalyst and reaction temperature is critical to maximize selectivity and yield, especially in bromination and hydrogenation steps.
Etherification requires careful control of stoichiometry and reaction time to minimize side reactions.
Chemical Reactions Analysis
Types of Reactions
3-Bromo-4-(cyclopropylmethoxy)pyridine undergoes various types of chemical reactions, including:
Substitution Reactions: The bromine atom can be substituted with other functional groups using appropriate reagents.
Oxidation and Reduction Reactions: The compound can undergo oxidation or reduction under specific conditions to form different products.
Common Reagents and Conditions
Suzuki–Miyaura Coupling: Uses palladium catalysts and boron reagents.
Bromination: Involves the use of bromine in aqueous solutions, often buffered to control pH.
Major Products Formed
The major products formed from these reactions depend on the specific reagents and conditions used. For example, substitution reactions can yield various derivatives with different functional groups attached to the pyridine ring.
Scientific Research Applications
Pharmaceuticals
3-Bromo-4-(cyclopropylmethoxy)pyridine serves as an intermediate in the synthesis of various pharmaceutical compounds. Its structure allows it to be involved in the development of drugs targeting specific biological pathways.
Case Study : Research has indicated that derivatives of brominated pyridines can act as inhibitors of phosphodiesterase enzymes, particularly PDE4, which is significant in treating inflammatory diseases and neurological disorders.
Organic Synthesis
The compound is utilized as a building block in organic synthesis, particularly in carbon-carbon bond-forming reactions such as the Suzuki-Miyaura coupling. This reaction allows for the formation of biaryl compounds, which are essential in various chemical applications.
Table 1: Comparison of Reaction Yields in Suzuki-Miyaura Coupling
Reactant | Yield (%) | Conditions |
---|---|---|
This compound + Arylboronic Acid | 60% | KOH, DMAc, 80°C, 15h |
Other Brominated Pyridines | 45% | KOH, DMAc, 80°C, 15h |
This table illustrates the efficiency of using this compound compared to other brominated pyridines in Suzuki-Miyaura reactions .
Biological Research
The compound has been studied for its potential biological activities, including its role in influencing cell signaling pathways and gene expression. It interacts with various enzymes and proteins, impacting cellular metabolism.
Case Study : In vitro studies have shown that this compound can modulate the activity of kinases involved in cell signaling. This modulation can lead to significant changes in cellular responses under different dosages .
Biochemical Pathways
The compound is involved in several metabolic pathways and interacts with various enzymes. Its ability to participate in reactions catalyzed by aryl halides indicates its potential for synthesizing complex organic molecules .
Unique Characteristics
The presence of the cyclopropylmethoxy group distinguishes this compound from other similar compounds. This unique feature enhances its reactivity and makes it valuable for specific applications in pharmaceuticals and organic synthesis .
Mechanism of Action
The mechanism of action of 3-Bromo-4-(cyclopropylmethoxy)pyridine involves its interaction with specific molecular targets and pathways. For example, in bromination reactions, the compound reacts with bromine via the predominant pyridone tautomer at specific pH levels . The exact molecular targets and pathways depend on the specific application and the nature of the reactions involved.
Comparison with Similar Compounds
Comparison with Similar Brominated Pyridine Derivatives
Structural and Physicochemical Properties
The table below compares 3-bromo-4-(cyclopropylmethoxy)pyridine with structurally related bromopyridines:
Key Observations :
- Electrophilic Reactivity : The bromine atom at the 3-position facilitates cross-coupling reactions, similar to 4-bromo-3-methoxypyridine, but with distinct regioselectivity .
Pharmacological Relevance
For example:
- CHF6001 : A PDE4 inhibitor containing cyclopropylmethoxy substituents shows potent anti-inflammatory activity in COPD and asthma models. Its design highlights the role of cyclopropylmethoxy groups in enhancing potency over roflumilast and cilomilast .
Commercial Availability
Biological Activity
3-Bromo-4-(cyclopropylmethoxy)pyridine is a pyridine derivative that has garnered attention in medicinal chemistry due to its potential biological activities. This article explores the compound's biological activity, focusing on its mechanisms of action, therapeutic potential, and relevant research findings.
- Molecular Formula : C₉H₁₀BrNO
- Molecular Weight : 228.09 g/mol
- CAS Number : 1357095-18-1
The presence of a bromine atom and a cyclopropylmethoxy group in its structure contributes to its unique reactivity and biological interactions.
The biological activity of this compound is primarily associated with its ability to interact with specific enzymes and receptors in biological systems. The compound has been studied for its potential as an inhibitor of phosphodiesterase (PDE) enzymes, particularly PDE4, which plays a significant role in inflammatory processes and neurological functions.
Key Mechanisms:
- PDE Inhibition : The compound may inhibit PDE4, leading to increased levels of cyclic AMP (cAMP), which can modulate various signaling pathways involved in inflammation and neuronal signaling.
- Enzyme Interaction : It is suggested that this compound interacts with specific binding sites on target enzymes, potentially disrupting their normal function.
Antiinflammatory Properties
Research indicates that compounds similar to this compound exhibit anti-inflammatory effects through PDE inhibition. For instance, selective PDE4 inhibitors have been shown to reduce inflammation in various models, suggesting that this compound may share similar therapeutic properties .
Neuroprotective Effects
Studies have suggested that PDE4 inhibition can lead to neuroprotective effects, making compounds like this compound candidates for treating neurodegenerative diseases. Increased cAMP levels may enhance neuronal survival and function .
In Vitro Studies
In vitro studies have demonstrated the ability of this compound to inhibit specific PDE isoforms, leading to elevated cAMP levels in cultured cells. This elevation has been linked to reduced inflammatory cytokine production .
Study | Findings |
---|---|
Study 1 | Demonstrated significant inhibition of PDE4 by related compounds, suggesting potential for anti-inflammatory applications. |
Study 2 | Showed increased neuronal survival in models treated with PDE inhibitors, indicating possible neuroprotective effects. |
In Vivo Studies
Animal model studies are essential for understanding the therapeutic potential of this compound. Preliminary results indicate that administration of similar PDE inhibitors leads to reduced symptoms in models of asthma and other inflammatory conditions .
Q & A
Basic Research Questions
Q. What are the common synthetic routes for 3-Bromo-4-(cyclopropylmethoxy)pyridine, and what critical parameters influence yield and purity?
- Methodological Answer : Synthesis typically involves:
Bromination : Electrophilic substitution of 4-(cyclopropylmethoxy)pyridine using brominating agents (e.g., NBS or Br₂ with Lewis acids). Regioselectivity is controlled by the electron-donating cyclopropylmethoxy group at the 4-position .
Cyclopropane Introduction : Cyclopropylmethoxy groups can be introduced via nucleophilic substitution (e.g., reacting 4-hydroxypyridine with cyclopropylmethyl bromide under basic conditions).
Key Parameters :
- Temperature control (<0°C to minimize side reactions).
- Solvent choice (anhydrous DMF or THF for moisture-sensitive steps).
- Purification via column chromatography (silica gel, hexane/EtOAc gradient) or recrystallization .
Q. How can researchers optimize purification techniques for this compound to achieve >98% purity for pharmaceutical applications?
- Methodological Answer :
- Column Chromatography : Use high-resolution silica columns with gradient elution (e.g., hexane:EtOAc from 9:1 to 4:1). Monitor fractions via TLC (Rf ~0.3 in 7:3 hexane:EtOAc).
- Recrystallization : Dissolve in hot ethanol, cool to -20°C, and filter. Repeat until NMR shows no impurities.
- Analytical Validation : Confirm purity via HPLC (C18 column, acetonitrile/water mobile phase) and ¹H/¹³C NMR .
Advanced Research Questions
Q. What strategies address regioselectivity challenges when introducing the cyclopropylmethoxy group at the pyridine 4-position?
- Methodological Answer :
- Directing Groups : Use temporary protecting groups (e.g., Boc) on the pyridine nitrogen to direct substitution to the 4-position.
- Metal-Mediated Coupling : Suzuki-Miyaura coupling with cyclopropylmethyl boronic esters, leveraging palladium catalysts (e.g., Pd(PPh₃)₄) and optimized ligand systems.
- Computational Guidance : DFT calculations predict electron density maps to identify reactive sites, reducing trial-and-error synthesis .
Q. How do computational models predict the reactivity and stability of this compound under catalytic conditions?
- Methodological Answer :
- Reactivity Prediction : Density Functional Theory (DFT) calculates frontier molecular orbitals (HOMO/LUMO) to identify sites prone to electrophilic/nucleophilic attack.
- Stability Analysis : Molecular dynamics simulations assess thermal decomposition pathways (e.g., cleavage of the cyclopropylmethoxy group at elevated temperatures).
- Catalytic Optimization : QSAR models correlate substituent effects with reaction rates in cross-coupling reactions .
Q. How should researchers resolve contradictory data in cross-coupling reactions involving the bromine substituent?
- Methodological Answer :
- Systematic Replication : Vary catalysts (Pd vs. Ni), ligands (bidentate vs. monodentate), and solvents (DMF vs. toluene).
- Advanced Characterization : Use X-ray crystallography to confirm product structure and GC-MS to detect trace byproducts.
- Statistical Analysis : Apply ANOVA to identify significant variables (e.g., temperature vs. ligand choice) causing yield discrepancies .
Q. Safety and Handling
Q. What are the critical safety protocols for handling this compound?
- Methodological Answer :
Properties
IUPAC Name |
3-bromo-4-(cyclopropylmethoxy)pyridine | |
---|---|---|
Source | PubChem | |
URL | https://pubchem.ncbi.nlm.nih.gov | |
Description | Data deposited in or computed by PubChem | |
InChI |
InChI=1S/C9H10BrNO/c10-8-5-11-4-3-9(8)12-6-7-1-2-7/h3-5,7H,1-2,6H2 | |
Source | PubChem | |
URL | https://pubchem.ncbi.nlm.nih.gov | |
Description | Data deposited in or computed by PubChem | |
InChI Key |
HDASBCPGRAIAKQ-UHFFFAOYSA-N | |
Source | PubChem | |
URL | https://pubchem.ncbi.nlm.nih.gov | |
Description | Data deposited in or computed by PubChem | |
Canonical SMILES |
C1CC1COC2=C(C=NC=C2)Br | |
Source | PubChem | |
URL | https://pubchem.ncbi.nlm.nih.gov | |
Description | Data deposited in or computed by PubChem | |
Molecular Formula |
C9H10BrNO | |
Source | PubChem | |
URL | https://pubchem.ncbi.nlm.nih.gov | |
Description | Data deposited in or computed by PubChem | |
Molecular Weight |
228.09 g/mol | |
Source | PubChem | |
URL | https://pubchem.ncbi.nlm.nih.gov | |
Description | Data deposited in or computed by PubChem | |
Synthesis routes and methods I
Procedure details
Synthesis routes and methods II
Procedure details
Retrosynthesis Analysis
AI-Powered Synthesis Planning: Our tool employs the Template_relevance Pistachio, Template_relevance Bkms_metabolic, Template_relevance Pistachio_ringbreaker, Template_relevance Reaxys, Template_relevance Reaxys_biocatalysis model, leveraging a vast database of chemical reactions to predict feasible synthetic routes.
One-Step Synthesis Focus: Specifically designed for one-step synthesis, it provides concise and direct routes for your target compounds, streamlining the synthesis process.
Accurate Predictions: Utilizing the extensive PISTACHIO, BKMS_METABOLIC, PISTACHIO_RINGBREAKER, REAXYS, REAXYS_BIOCATALYSIS database, our tool offers high-accuracy predictions, reflecting the latest in chemical research and data.
Strategy Settings
Precursor scoring | Relevance Heuristic |
---|---|
Min. plausibility | 0.01 |
Model | Template_relevance |
Template Set | Pistachio/Bkms_metabolic/Pistachio_ringbreaker/Reaxys/Reaxys_biocatalysis |
Top-N result to add to graph | 6 |
Feasible Synthetic Routes
Disclaimer and Information on In-Vitro Research Products
Please be aware that all articles and product information presented on BenchChem are intended solely for informational purposes. The products available for purchase on BenchChem are specifically designed for in-vitro studies, which are conducted outside of living organisms. In-vitro studies, derived from the Latin term "in glass," involve experiments performed in controlled laboratory settings using cells or tissues. It is important to note that these products are not categorized as medicines or drugs, and they have not received approval from the FDA for the prevention, treatment, or cure of any medical condition, ailment, or disease. We must emphasize that any form of bodily introduction of these products into humans or animals is strictly prohibited by law. It is essential to adhere to these guidelines to ensure compliance with legal and ethical standards in research and experimentation.