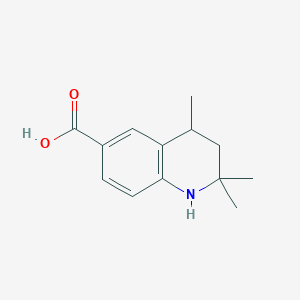
2,2,4-Trimethyl-1,2,3,4-tetrahydroquinoline-6-carboxylic acid
Overview
Description
2,2,4-Trimethyl-1,2,3,4-tetrahydroquinoline-6-carboxylic acid is a useful research compound. Its molecular formula is C13H17NO2 and its molecular weight is 219.28 g/mol. The purity is usually 95%.
BenchChem offers high-quality this compound suitable for many research applications. Different packaging options are available to accommodate customers' requirements. Please inquire for more information about this compound including the price, delivery time, and more detailed information at info@benchchem.com.
Mechanism of Action
Mode of Action
It is known that quinoline derivatives, such as this compound, have the potential to function as powerful antioxidants .
Biochemical Pathways
It is suggested that quinoline derivatives can potentially counteract oxidative damage, which could be significantly helpful in neuroprotection .
Pharmacokinetics
These properties are crucial in determining the bioavailability of the compound .
Result of Action
Based on its antioxidant potential, it may help in mitigating oxidative stress .
Action Environment
It is recommended to store the compound in a dark place, sealed in dry conditions, at room temperature for optimal stability .
Biochemical Analysis
Biochemical Properties
2,2,4-Trimethyl-1,2,3,4-tetrahydroquinoline-6-carboxylic acid plays a crucial role in biochemical reactions, particularly in its interactions with enzymes, proteins, and other biomolecules. It has been observed to interact with NADPH-generating enzymes and chaperones, enhancing their activity and contributing to the antioxidant defense system . The compound’s antioxidant properties are significant in reducing oxidative stress and normalizing chaperone activity, which is essential for maintaining cellular homeostasis .
Cellular Effects
The effects of this compound on various cell types and cellular processes are profound. It influences cell function by enhancing the antioxidant system, reducing oxidative stress, and normalizing chaperone activity . This compound also affects cell signaling pathways, gene expression, and cellular metabolism. For instance, it has been shown to upregulate the mRNA expression levels of antioxidant genes and factors such as Nrf2 and Foxo1, which play critical roles in cellular defense mechanisms .
Molecular Mechanism
At the molecular level, this compound exerts its effects through various mechanisms. It binds to NADPH-generating enzymes and chaperones, enhancing their activity and contributing to the antioxidant defense system . The compound also inhibits apoptosis by normalizing chaperone activity and reducing oxidative stress . Additionally, it upregulates the expression of antioxidant genes and factors, further enhancing its protective effects on cells .
Temporal Effects in Laboratory Settings
In laboratory settings, the effects of this compound change over time. The compound has been shown to be stable under various conditions, maintaining its antioxidant properties and effectiveness in reducing oxidative stress over extended periods . Long-term studies have demonstrated that it continues to enhance the antioxidant defense system and normalize chaperone activity, contributing to sustained cellular protection .
Dosage Effects in Animal Models
The effects of this compound vary with different dosages in animal models. Studies have shown that higher doses of the compound result in more significant reductions in oxidative stress and greater enhancements in antioxidant enzyme activities . At very high doses, there may be potential toxic or adverse effects, highlighting the importance of determining the optimal dosage for therapeutic applications .
Metabolic Pathways
This compound is involved in various metabolic pathways, particularly those related to the antioxidant defense system. It interacts with NADPH-generating enzymes, enhancing their activity and contributing to the reduction of oxidative stress . The compound’s involvement in these pathways is crucial for maintaining cellular homeostasis and protecting cells from oxidative damage .
Transport and Distribution
The transport and distribution of this compound within cells and tissues are essential for its effectiveness. The compound is transported via specific transporters and binding proteins, which facilitate its localization and accumulation in target tissues . This targeted distribution is crucial for maximizing its antioxidant effects and protecting cells from oxidative stress .
Subcellular Localization
This compound is localized in specific subcellular compartments, where it exerts its antioxidant effects. The compound’s targeting signals and post-translational modifications direct it to these compartments, ensuring its effective function . This subcellular localization is critical for its role in enhancing the antioxidant defense system and protecting cells from oxidative damage .
Properties
IUPAC Name |
2,2,4-trimethyl-3,4-dihydro-1H-quinoline-6-carboxylic acid | |
---|---|---|
Source | PubChem | |
URL | https://pubchem.ncbi.nlm.nih.gov | |
Description | Data deposited in or computed by PubChem | |
InChI |
InChI=1S/C13H17NO2/c1-8-7-13(2,3)14-11-5-4-9(12(15)16)6-10(8)11/h4-6,8,14H,7H2,1-3H3,(H,15,16) | |
Source | PubChem | |
URL | https://pubchem.ncbi.nlm.nih.gov | |
Description | Data deposited in or computed by PubChem | |
InChI Key |
QVFOSMHNHGVRJT-UHFFFAOYSA-N | |
Source | PubChem | |
URL | https://pubchem.ncbi.nlm.nih.gov | |
Description | Data deposited in or computed by PubChem | |
Canonical SMILES |
CC1CC(NC2=C1C=C(C=C2)C(=O)O)(C)C | |
Source | PubChem | |
URL | https://pubchem.ncbi.nlm.nih.gov | |
Description | Data deposited in or computed by PubChem | |
Molecular Formula |
C13H17NO2 | |
Source | PubChem | |
URL | https://pubchem.ncbi.nlm.nih.gov | |
Description | Data deposited in or computed by PubChem | |
Molecular Weight |
219.28 g/mol | |
Source | PubChem | |
URL | https://pubchem.ncbi.nlm.nih.gov | |
Description | Data deposited in or computed by PubChem | |
Retrosynthesis Analysis
AI-Powered Synthesis Planning: Our tool employs the Template_relevance Pistachio, Template_relevance Bkms_metabolic, Template_relevance Pistachio_ringbreaker, Template_relevance Reaxys, Template_relevance Reaxys_biocatalysis model, leveraging a vast database of chemical reactions to predict feasible synthetic routes.
One-Step Synthesis Focus: Specifically designed for one-step synthesis, it provides concise and direct routes for your target compounds, streamlining the synthesis process.
Accurate Predictions: Utilizing the extensive PISTACHIO, BKMS_METABOLIC, PISTACHIO_RINGBREAKER, REAXYS, REAXYS_BIOCATALYSIS database, our tool offers high-accuracy predictions, reflecting the latest in chemical research and data.
Strategy Settings
Precursor scoring | Relevance Heuristic |
---|---|
Min. plausibility | 0.01 |
Model | Template_relevance |
Template Set | Pistachio/Bkms_metabolic/Pistachio_ringbreaker/Reaxys/Reaxys_biocatalysis |
Top-N result to add to graph | 6 |
Feasible Synthetic Routes
Disclaimer and Information on In-Vitro Research Products
Please be aware that all articles and product information presented on BenchChem are intended solely for informational purposes. The products available for purchase on BenchChem are specifically designed for in-vitro studies, which are conducted outside of living organisms. In-vitro studies, derived from the Latin term "in glass," involve experiments performed in controlled laboratory settings using cells or tissues. It is important to note that these products are not categorized as medicines or drugs, and they have not received approval from the FDA for the prevention, treatment, or cure of any medical condition, ailment, or disease. We must emphasize that any form of bodily introduction of these products into humans or animals is strictly prohibited by law. It is essential to adhere to these guidelines to ensure compliance with legal and ethical standards in research and experimentation.