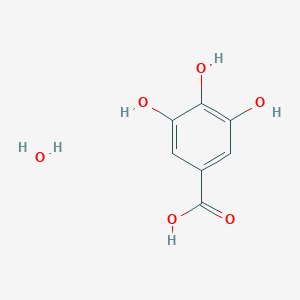
Gallic acid monohydrate
Overview
Description
Mechanism of Action
Target of Action
Gallic acid monohydrate is a phenolic acid that has significant importance due to its antioxidant and anti-cancer activities . It primarily targets the cell cycle, proliferation, migration, and expression of programmed death-ligand 1 (PD-L1) . These targets play a crucial role in the progression of tumors .
Mode of Action
This compound interacts with its targets by inducing uncontrolled cell cycle, proliferation, migration, and expression of PD-L1 . This interaction results in changes that aid in the progression of tumors . Additionally, it has been suggested that the gastroprotective activity of gallic acid may be related to antioxidant properties, immunomodulatory markers, Hsp70 and Bcl-2-associated X protein, and inhibition of mitochondrial apoptosis .
Biochemical Pathways
Gallic acid is formed from 3-dehydroshikimate by the action of the enzyme shikimate dehydrogenase to produce 3,5-didehydroshikimate . This compound then aromatizes . In metabolic disorders, gallic acid inhibits diet-induced hyperglycemia and hypertriglyceridemia, reduces the size of adipocytes, and protects pancreatic β-cells by inducing the expression of peroxisome proliferator-activated receptor-γ (PPAR-γ), a nuclear transcription factor that induces differentiation and insulin sensitivity in adipocytes .
Pharmacokinetics
Gallic acid exhibits slower absorption into the bloodstream, yielding less values of area under concentration–time curve as well as lower maximum blood concentration (Cmax) in myocardial infarcted rats than those in normal rats . Significant prolonged T1/2 and MRT as well as decreased CL were also registered in myocardial infarcted rats . Its pharmacokinetic features such as low absorption, poor bioavailability, and rapid elimination severely restrict its use .
Result of Action
The molecular and cellular effects of gallic acid’s action include antioxidant and anti-cancer activities . It can prevent cancer progression, cell invasion, and metastasis by targeting molecular pathways . It also has potent therapeutic effects on the non-steroidal anti-inflammatory drug (NSAID)-induced gastric mucosal damage by preventing oxidative stress and inhibiting the activation of the mitochondrial pathway of apoptosis in gastric mucosal cells .
Action Environment
The action, efficacy, and stability of gallic acid are influenced by environmental factors. For instance, hydration/dehydration behavior is very dependent on relative humidity and phase purity . Moreover, this compound is easily hygroscopic in humid air .
Biochemical Analysis
Biochemical Properties
Gallic acid monohydrate plays a crucial role in various biochemical reactions. It is involved in the synthesis of plant hydrolysable tannins and acts as a primary anti-inflammatory and cardio-protective agent. The compound interacts with several enzymes, proteins, and other biomolecules. For instance, shikimate dehydrogenase, an enzyme in the shikimate pathway, is essential for the production of this compound. This enzyme catalyzes the dehydrogenation of 3-dehydroshikimate to produce this compound . Additionally, this compound can interact with proteins through hydrogen bonding and hydrophobic interactions, influencing their structure and function.
Cellular Effects
This compound exerts various effects on different cell types and cellular processes. It has been shown to influence cell signaling pathways, gene expression, and cellular metabolism. For example, this compound can modulate the expression of genes involved in oxidative stress response, apoptosis, and inflammation. It also affects cell signaling pathways such as the NF-κB and MAPK pathways, leading to changes in cell proliferation, differentiation, and survival . Furthermore, this compound can alter cellular metabolism by affecting the activity of key metabolic enzymes and pathways.
Molecular Mechanism
The molecular mechanism of action of this compound involves several binding interactions with biomolecules, enzyme inhibition or activation, and changes in gene expression. This compound can bind to proteins and enzymes, altering their activity and function. For instance, it has been shown to inhibit the activity of certain enzymes involved in inflammation and oxidative stress, such as cyclooxygenase and lipoxygenase . Additionally, this compound can modulate gene expression by interacting with transcription factors and signaling molecules, leading to changes in the expression of genes involved in various cellular processes.
Temporal Effects in Laboratory Settings
In laboratory settings, the effects of this compound can change over time. The compound’s stability and degradation are important factors to consider. This compound is relatively stable under normal laboratory conditions, but it can degrade over time, especially when exposed to light and heat . Long-term studies have shown that this compound can have sustained effects on cellular function, including prolonged antioxidant and anti-inflammatory activities. The extent and duration of these effects can vary depending on the experimental conditions and the specific cell type or tissue being studied.
Dosage Effects in Animal Models
The effects of this compound can vary with different dosages in animal models. Studies have shown that low to moderate doses of this compound can have beneficial effects, such as reducing oxidative stress and inflammation, and improving cardiovascular health . At high doses, this compound can exhibit toxic or adverse effects, including hepatotoxicity and nephrotoxicity. The threshold for these effects can vary depending on the animal model and the specific experimental conditions.
Metabolic Pathways
This compound is involved in several metabolic pathways, including the shikimate pathway, which is essential for the synthesis of aromatic amino acids and other secondary metabolites. The compound interacts with enzymes such as shikimate dehydrogenase and 3-dehydroshikimate dehydratase, influencing the metabolic flux and levels of metabolites in these pathways . Additionally, this compound can affect the activity of other metabolic enzymes, leading to changes in the overall metabolic profile of cells and tissues.
Transport and Distribution
Within cells and tissues, this compound is transported and distributed through various mechanisms. It can interact with transporters and binding proteins, influencing its localization and accumulation. For example, this compound can be transported across cell membranes by specific transporters, such as the monocarboxylate transporter family . Once inside the cell, it can bind to proteins and other biomolecules, affecting its distribution and activity.
Subcellular Localization
The subcellular localization of this compound can influence its activity and function. The compound can be found in various cellular compartments, including the cytoplasm, nucleus, and mitochondria . Its localization is often determined by targeting signals and post-translational modifications that direct it to specific organelles. For instance, this compound can accumulate in the mitochondria, where it exerts its antioxidant effects by scavenging reactive oxygen species and protecting mitochondrial function.
Preparation Methods
Synthetic Routes and Reaction Conditions: Gallic acid can be synthesized through the hydrolysis of gallotannins, which are found in plants like Terminalia bellirica. The hydrolysis can be performed under acidic or alkaline conditions . Another method involves the use of macroporous resin column chromatography followed by preparative high-performance liquid chromatography (HPLC) to isolate and purify gallic acid .
Industrial Production Methods: In industrial settings, gallic acid is often produced by the hydrolysis of tannins extracted from plant sources. The process involves heating the tannins with sulfuric acid, which breaks them down into gallic acid and glucose .
Types of Reactions:
Substitution: The hydroxyl groups in gallic acid can participate in esterification reactions with alcohols to form esters.
Common Reagents and Conditions:
Oxidizing Agents: Arsenic acid, permanganate, persulfate, iodine.
Reducing Agents: Gallic acid itself acts as a reducing agent.
Esterification Conditions: Azeotropic esterification or Fischer method using alcohol and hydrochloric acid.
Major Products:
Ellagic Acid: Formed through oxidation.
Pyrogallol: Formed through decarboxylation at high temperatures.
Scientific Research Applications
Gallic acid (hydrate) has a wide range of applications in scientific research:
Comparison with Similar Compounds
Benzoic Acid: A simple aromatic carboxylic acid with antimicrobial properties.
Phenol: A hydroxyl group attached to a benzene ring, known for its antiseptic properties.
Pyrogallol: A trihydroxybenzene similar to gallic acid but without the carboxyl group.
Uniqueness of Gallic Acid: Gallic acid is unique due to its three hydroxyl groups and one carboxyl group, which confer strong antioxidant properties. Its ability to undergo various chemical reactions and its wide range of applications in different fields make it a versatile compound .
Properties
IUPAC Name |
3,4,5-trihydroxybenzoic acid;hydrate | |
---|---|---|
Source | PubChem | |
URL | https://pubchem.ncbi.nlm.nih.gov | |
Description | Data deposited in or computed by PubChem | |
InChI |
InChI=1S/C7H6O5.H2O/c8-4-1-3(7(11)12)2-5(9)6(4)10;/h1-2,8-10H,(H,11,12);1H2 | |
Source | PubChem | |
URL | https://pubchem.ncbi.nlm.nih.gov | |
Description | Data deposited in or computed by PubChem | |
InChI Key |
IUTKPPDDLYYMBE-UHFFFAOYSA-N | |
Source | PubChem | |
URL | https://pubchem.ncbi.nlm.nih.gov | |
Description | Data deposited in or computed by PubChem | |
Canonical SMILES |
C1=C(C=C(C(=C1O)O)O)C(=O)O.O | |
Source | PubChem | |
URL | https://pubchem.ncbi.nlm.nih.gov | |
Description | Data deposited in or computed by PubChem | |
Molecular Formula |
C7H8O6 | |
Source | PubChem | |
URL | https://pubchem.ncbi.nlm.nih.gov | |
Description | Data deposited in or computed by PubChem | |
Molecular Weight |
188.13 g/mol | |
Source | PubChem | |
URL | https://pubchem.ncbi.nlm.nih.gov | |
Description | Data deposited in or computed by PubChem | |
CAS No. |
5995-86-8 | |
Record name | 3,4,5-Trihydroxybenzoic acid hydrate (1:1) | |
Source | CAS Common Chemistry | |
URL | https://commonchemistry.cas.org/detail?cas_rn=5995-86-8 | |
Description | CAS Common Chemistry is an open community resource for accessing chemical information. Nearly 500,000 chemical substances from CAS REGISTRY cover areas of community interest, including common and frequently regulated chemicals, and those relevant to high school and undergraduate chemistry classes. This chemical information, curated by our expert scientists, is provided in alignment with our mission as a division of the American Chemical Society. | |
Explanation | The data from CAS Common Chemistry is provided under a CC-BY-NC 4.0 license, unless otherwise stated. | |
Record name | Gallic acid monohydrate | |
Source | ChemIDplus | |
URL | https://pubchem.ncbi.nlm.nih.gov/substance/?source=chemidplus&sourceid=0005995868 | |
Description | ChemIDplus is a free, web search system that provides access to the structure and nomenclature authority files used for the identification of chemical substances cited in National Library of Medicine (NLM) databases, including the TOXNET system. | |
Record name | GALLIC ACID MONOHYDRATE | |
Source | FDA Global Substance Registration System (GSRS) | |
URL | https://gsrs.ncats.nih.gov/ginas/app/beta/substances/48339473OT | |
Description | The FDA Global Substance Registration System (GSRS) enables the efficient and accurate exchange of information on what substances are in regulated products. Instead of relying on names, which vary across regulatory domains, countries, and regions, the GSRS knowledge base makes it possible for substances to be defined by standardized, scientific descriptions. | |
Explanation | Unless otherwise noted, the contents of the FDA website (www.fda.gov), both text and graphics, are not copyrighted. They are in the public domain and may be republished, reprinted and otherwise used freely by anyone without the need to obtain permission from FDA. Credit to the U.S. Food and Drug Administration as the source is appreciated but not required. | |
Retrosynthesis Analysis
AI-Powered Synthesis Planning: Our tool employs the Template_relevance Pistachio, Template_relevance Bkms_metabolic, Template_relevance Pistachio_ringbreaker, Template_relevance Reaxys, Template_relevance Reaxys_biocatalysis model, leveraging a vast database of chemical reactions to predict feasible synthetic routes.
One-Step Synthesis Focus: Specifically designed for one-step synthesis, it provides concise and direct routes for your target compounds, streamlining the synthesis process.
Accurate Predictions: Utilizing the extensive PISTACHIO, BKMS_METABOLIC, PISTACHIO_RINGBREAKER, REAXYS, REAXYS_BIOCATALYSIS database, our tool offers high-accuracy predictions, reflecting the latest in chemical research and data.
Strategy Settings
Precursor scoring | Relevance Heuristic |
---|---|
Min. plausibility | 0.01 |
Model | Template_relevance |
Template Set | Pistachio/Bkms_metabolic/Pistachio_ringbreaker/Reaxys/Reaxys_biocatalysis |
Top-N result to add to graph | 6 |
Feasible Synthetic Routes
Disclaimer and Information on In-Vitro Research Products
Please be aware that all articles and product information presented on BenchChem are intended solely for informational purposes. The products available for purchase on BenchChem are specifically designed for in-vitro studies, which are conducted outside of living organisms. In-vitro studies, derived from the Latin term "in glass," involve experiments performed in controlled laboratory settings using cells or tissues. It is important to note that these products are not categorized as medicines or drugs, and they have not received approval from the FDA for the prevention, treatment, or cure of any medical condition, ailment, or disease. We must emphasize that any form of bodily introduction of these products into humans or animals is strictly prohibited by law. It is essential to adhere to these guidelines to ensure compliance with legal and ethical standards in research and experimentation.