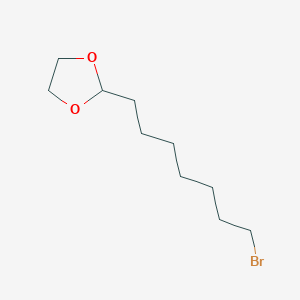
1,3-Dioxolane, 2-(7-bromoheptyl)-
- Click on QUICK INQUIRY to receive a quote from our team of experts.
- With the quality product at a COMPETITIVE price, you can focus more on your research.
Overview
Description
1,3-Dioxolane, 2-(7-bromoheptyl)- is a chemical compound with the molecular formula C10H19BrO2 and a molecular weight of 251.16 g/mol . It is a derivative of 1,3-dioxolane, a heterocyclic acetal with a five-membered ring containing two oxygen atoms. The compound is characterized by the presence of a 7-bromoheptyl group attached to the 2-position of the 1,3-dioxolane ring.
Preparation Methods
Synthetic Routes and Reaction Conditions
1,3-Dioxolane, 2-(7-bromoheptyl)- can be synthesized through the acetalization of carbonyl compounds with 1,2-ethanediol in the presence of a Brönsted or Lewis acid catalyst . The reaction typically involves the use of toluenesulfonic acid as a catalyst in refluxing toluene, allowing for the continuous removal of water from the reaction mixture using a Dean-Stark apparatus . Other catalysts such as zirconium tetrachloride (ZrCl4) and tetrabutylammonium tribromide can also be used for acetalization under mild conditions .
Industrial Production Methods
Industrial production of 1,3-dioxolane derivatives often involves the use of large-scale reactors and continuous flow processes to ensure efficient synthesis and high yields. The use of molecular sieves or orthoesters can help in the effective removal of water, thereby driving the reaction to completion .
Chemical Reactions Analysis
Types of Reactions
1,3-Dioxolane, 2-(7-bromoheptyl)- undergoes various chemical reactions, including:
Oxidation: The compound can be oxidized using agents such as potassium permanganate (KMnO4) and osmium tetroxide (OsO4).
Common Reagents and Conditions
Oxidation: KMnO4, OsO4, and chromium trioxide (CrO3) in pyridine.
Reduction: LiAlH4, NaBH4, and hydrogenation with nickel or rhodium catalysts.
Substitution: Nucleophiles like RLi, RMgX, and RCuLi in the presence of bases such as LDA or t-BuOK.
Major Products Formed
Oxidation: Formation of carboxylic acids or ketones.
Reduction: Formation of alcohols.
Substitution: Formation of various substituted derivatives depending on the nucleophile used.
Scientific Research Applications
1,3-Dioxolane, 2-(7-bromoheptyl)- has several applications in scientific research:
Chemistry: Used as a building block in organic synthesis and as a protecting group for carbonyl compounds.
Biology: Employed in the synthesis of biologically active molecules and as a precursor for drug development.
Medicine: Investigated for its potential use in the development of pharmaceuticals and therapeutic agents.
Industry: Utilized in the production of polymers and as a solvent in various industrial processes.
Mechanism of Action
The mechanism of action of 1,3-dioxolane, 2-(7-bromoheptyl)- involves its ability to undergo ring-opening polymerization and substitution reactions. The compound can act as a monomer in the formation of polymers, and its bromine atom can participate in nucleophilic substitution reactions . The molecular targets and pathways involved depend on the specific application and the nature of the substituents introduced during the reactions.
Comparison with Similar Compounds
1,3-Dioxolane, 2-(7-bromoheptyl)- can be compared with other similar compounds such as:
1,3-Dioxane: A six-membered ring analog with similar reactivity but different ring strain and stability.
Tetrahydrofuran (THF): A five-membered ring ether with similar solvent properties but lacking the acetal functionality.
γ-Valerolactone (GVL): A green solvent with similar polar aprotic properties but different structural features.
The uniqueness of 1,3-dioxolane, 2-(7-bromoheptyl)- lies in its specific substitution pattern and the presence of the bromine atom, which allows for diverse chemical transformations and applications.
Biological Activity
Chemical Structure and Properties
1,3-Dioxolane, 2-(7-bromoheptyl)- is a cyclic ether with the molecular formula C10H19BrO2. The compound features a dioxolane ring substituted with a bromoheptyl group, which influences its biological activity. This compound belongs to a class of compounds known for their diverse biological activities, including antimicrobial and anticancer properties.
Antimicrobial Properties
Research indicates that various derivatives of 1,3-dioxolanes exhibit significant antibacterial and antifungal activities. A study synthesized several 1,3-dioxolane derivatives and tested their efficacy against various bacterial strains:
-
Bacterial Strains Tested:
- Staphylococcus aureus
- Staphylococcus epidermidis
- Enterococcus faecalis
- Pseudomonas aeruginosa
-
Antifungal Strain Tested:
- Candida albicans
The results demonstrated that most synthesized compounds exhibited excellent antifungal activity against C. albicans and significant antibacterial activity against S. aureus and S. epidermidis, with Minimum Inhibitory Concentration (MIC) values ranging from 625 to 1250 µg/mL for certain derivatives. Notably, the compound with the bromoheptyl substitution may enhance these activities due to its structural properties .
Anticancer Activity
1,3-Dioxolanes have also been explored for their potential as anticancer agents . Some studies have indicated that compounds containing the dioxolane structure can inhibit cancer cell proliferation and induce apoptosis. For instance, derivatives were tested in vitro for their cytotoxic effects on various cancer cell lines, showing promising results comparable to established chemotherapeutic agents .
The mechanisms by which 1,3-dioxolane derivatives exert their biological effects include:
- Cell Membrane Disruption: The lipophilic nature of the bromoheptyl group may facilitate penetration into bacterial membranes, leading to cell lysis.
- Enzyme Inhibition: Some studies suggest that these compounds can inhibit key enzymes involved in cellular metabolism in both bacteria and cancer cells.
- Signal Pathway Interference: Research has indicated potential interference with signaling pathways critical for cell survival and proliferation in cancer cells .
Case Study 1: Antifungal Activity
A study focused on the antifungal properties of synthesized dioxolane derivatives highlighted that compounds with specific substituents showed enhanced activity against C. albicans. The structure-activity relationship (SAR) analysis revealed that longer alkyl chains increased antifungal potency.
Case Study 2: Anticancer Screening
In another study, a series of dioxolane derivatives were screened against different cancer cell lines. The results indicated that several compounds exhibited IC50 values lower than those of established anticancer drugs, suggesting a potential for development into new therapeutic agents .
Comparative Table of Biological Activities
Compound | Antibacterial Activity (MIC µg/mL) | Antifungal Activity (MIC µg/mL) | Notable Effects |
---|---|---|---|
1,3-Dioxolane Derivative A | 625 | >1000 | Effective against S. aureus |
1,3-Dioxolane Derivative B | 1250 | <500 | Effective against C. albicans |
1,3-Dioxolane, 2-(7-bromoheptyl) | TBD | TBD | Potentially enhanced activity due to bromoheptyl group |
Properties
CAS No. |
92540-32-4 |
---|---|
Molecular Formula |
C10H19BrO2 |
Molecular Weight |
251.16 g/mol |
IUPAC Name |
2-(7-bromoheptyl)-1,3-dioxolane |
InChI |
InChI=1S/C10H19BrO2/c11-7-5-3-1-2-4-6-10-12-8-9-13-10/h10H,1-9H2 |
InChI Key |
ZIHSCVYGRZCQJR-UHFFFAOYSA-N |
Canonical SMILES |
C1COC(O1)CCCCCCCBr |
Origin of Product |
United States |
Disclaimer and Information on In-Vitro Research Products
Please be aware that all articles and product information presented on BenchChem are intended solely for informational purposes. The products available for purchase on BenchChem are specifically designed for in-vitro studies, which are conducted outside of living organisms. In-vitro studies, derived from the Latin term "in glass," involve experiments performed in controlled laboratory settings using cells or tissues. It is important to note that these products are not categorized as medicines or drugs, and they have not received approval from the FDA for the prevention, treatment, or cure of any medical condition, ailment, or disease. We must emphasize that any form of bodily introduction of these products into humans or animals is strictly prohibited by law. It is essential to adhere to these guidelines to ensure compliance with legal and ethical standards in research and experimentation.