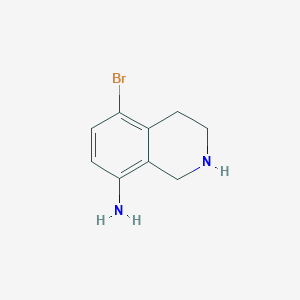
5-Bromo-1,2,3,4-tetrahydroisoquinolin-8-amine
Overview
Description
5-Bromo-1,2,3,4-tetrahydroisoquinolin-8-amine: is a chemical compound with the molecular formula C9H11BrN2 . It is a derivative of tetrahydroisoquinoline, a class of compounds known for their diverse biological activities. This compound is of interest in medicinal chemistry due to its potential therapeutic applications .
Biochemical Analysis
Biochemical Properties
5-Bromo-1,2,3,4-tetrahydroisoquinolin-8-amine plays a significant role in biochemical reactions. It interacts with various enzymes, proteins, and other biomolecules. For instance, it has been observed to interact with certain isoquinoline alkaloids, which are known for their biological activities against pathogens and neurodegenerative disorders . The nature of these interactions often involves binding to active sites of enzymes or receptors, thereby modulating their activity.
Cellular Effects
The effects of this compound on cells and cellular processes are profound. This compound influences cell function by affecting cell signaling pathways, gene expression, and cellular metabolism. For example, it can modulate the activity of signaling pathways that are crucial for cell survival and proliferation . Additionally, changes in gene expression induced by this compound can lead to alterations in cellular metabolism, impacting the overall function of the cell.
Molecular Mechanism
At the molecular level, this compound exerts its effects through various mechanisms. It can bind to specific biomolecules, leading to enzyme inhibition or activation. This binding can result in changes in gene expression, which in turn affects cellular functions . The compound’s ability to interact with different molecular targets makes it a versatile agent in biochemical research.
Temporal Effects in Laboratory Settings
The stability and degradation of this compound over time are crucial factors in laboratory settings. This compound is typically stored in dark places under inert atmospheres at temperatures between 2-8°C to maintain its stability . Over time, any degradation products formed can influence the long-term effects on cellular function observed in both in vitro and in vivo studies.
Dosage Effects in Animal Models
The effects of this compound vary with different dosages in animal models. At lower doses, the compound may exhibit beneficial effects, while at higher doses, toxic or adverse effects may be observed . Understanding the dosage thresholds is essential for determining the therapeutic window and potential side effects of this compound.
Metabolic Pathways
This compound is involved in various metabolic pathways. It interacts with enzymes and cofactors that facilitate its metabolism. These interactions can affect metabolic flux and the levels of metabolites within the cell . The study of these pathways provides insights into the compound’s pharmacokinetics and pharmacodynamics.
Transport and Distribution
The transport and distribution of this compound within cells and tissues are mediated by specific transporters and binding proteins. These interactions influence the localization and accumulation of the compound within different cellular compartments . Understanding these processes is crucial for determining the bioavailability and efficacy of the compound.
Subcellular Localization
The subcellular localization of this compound affects its activity and function. Targeting signals and post-translational modifications can direct the compound to specific compartments or organelles within the cell . This localization is essential for its interaction with molecular targets and the subsequent biological effects.
Preparation Methods
Synthetic Routes and Reaction Conditions: The synthesis of 5-Bromo-1,2,3,4-tetrahydroisoquinolin-8-amine typically involves the bromination of 1,2,3,4-tetrahydroisoquinoline. One common method is the Bischler-Napieralski reaction, which involves the cyclization of an N-acyl derivative of β-phenylethylamine in the presence of a dehydrating agent such as phosphorus oxychloride (POCl3), phosphorus pentoxide (P2O5), or zinc chloride (ZnCl2) .
Industrial Production Methods: Industrial production methods for this compound are not well-documented in the literature. the general approach would involve scaling up the laboratory synthesis methods, ensuring the reaction conditions are optimized for large-scale production.
Chemical Reactions Analysis
Types of Reactions: 5-Bromo-1,2,3,4-tetrahydroisoquinolin-8-amine can undergo various chemical reactions, including:
Oxidation: This compound can be oxidized to form corresponding quinoline derivatives.
Reduction: Reduction reactions can convert it into different tetrahydroisoquinoline derivatives.
Substitution: The bromine atom can be substituted with other functional groups using nucleophilic substitution reactions.
Common Reagents and Conditions:
Oxidation: Common oxidizing agents include potassium permanganate (KMnO4) and chromium trioxide (CrO3).
Reduction: Reducing agents such as sodium borohydride (NaBH4) or catalytic hydrogenation can be used.
Substitution: Nucleophiles like sodium azide (NaN3) or thiols (RSH) can be employed for substitution reactions.
Major Products: The major products formed from these reactions depend on the specific reagents and conditions used. For example, oxidation may yield quinoline derivatives, while reduction can produce various tetrahydroisoquinoline derivatives .
Scientific Research Applications
Chemistry: In chemistry, 5-Bromo-1,2,3,4-tetrahydroisoquinolin-8-amine is used as a building block for the synthesis of more complex molecules. Its unique structure allows for the creation of diverse chemical libraries for drug discovery .
Biology and Medicine: This compound has shown potential in medicinal chemistry for the development of drugs targeting neurological disorders and infectious diseases. Its derivatives have been studied for their activity against various pathogens and neurodegenerative conditions .
Industry: In the industrial sector, this compound can be used in the synthesis of specialty chemicals and materials. Its bromine functionality makes it a valuable intermediate for the production of flame retardants and other brominated compounds .
Mechanism of Action
The mechanism of action of 5-Bromo-1,2,3,4-tetrahydroisoquinolin-8-amine is not fully understood. it is believed to interact with specific molecular targets and pathways involved in neurological and infectious diseases. The compound may exert its effects by modulating neurotransmitter systems or inhibiting the growth of pathogens .
Comparison with Similar Compounds
1,2,3,4-Tetrahydroisoquinoline: A parent compound with a similar structure but without the bromine atom.
5-Bromo-1,2,3,4-tetrahydroisoquinoline: Similar to 5-Bromo-1,2,3,4-tetrahydroisoquinolin-8-amine but lacks the amine group at the 8th position.
2-Boc-5-bromo-1,2,3,4-tetrahydroisoquinolin-8-amine: A derivative with a tert-butoxycarbonyl (Boc) protecting group.
Uniqueness: this compound is unique due to the presence of both the bromine atom and the amine group, which confer distinct chemical reactivity and biological activity. This combination allows for versatile chemical modifications and potential therapeutic applications .
Properties
IUPAC Name |
5-bromo-1,2,3,4-tetrahydroisoquinolin-8-amine | |
---|---|---|
Source | PubChem | |
URL | https://pubchem.ncbi.nlm.nih.gov | |
Description | Data deposited in or computed by PubChem | |
InChI |
InChI=1S/C9H11BrN2/c10-8-1-2-9(11)7-5-12-4-3-6(7)8/h1-2,12H,3-5,11H2 | |
Source | PubChem | |
URL | https://pubchem.ncbi.nlm.nih.gov | |
Description | Data deposited in or computed by PubChem | |
InChI Key |
ZCXVHSIERUIKMS-UHFFFAOYSA-N | |
Source | PubChem | |
URL | https://pubchem.ncbi.nlm.nih.gov | |
Description | Data deposited in or computed by PubChem | |
Canonical SMILES |
C1CNCC2=C(C=CC(=C21)Br)N | |
Source | PubChem | |
URL | https://pubchem.ncbi.nlm.nih.gov | |
Description | Data deposited in or computed by PubChem | |
Molecular Formula |
C9H11BrN2 | |
Source | PubChem | |
URL | https://pubchem.ncbi.nlm.nih.gov | |
Description | Data deposited in or computed by PubChem | |
DSSTOX Substance ID |
DTXSID30733334 | |
Record name | 5-Bromo-1,2,3,4-tetrahydroisoquinolin-8-amine | |
Source | EPA DSSTox | |
URL | https://comptox.epa.gov/dashboard/DTXSID30733334 | |
Description | DSSTox provides a high quality public chemistry resource for supporting improved predictive toxicology. | |
Molecular Weight |
227.10 g/mol | |
Source | PubChem | |
URL | https://pubchem.ncbi.nlm.nih.gov | |
Description | Data deposited in or computed by PubChem | |
CAS No. |
1260779-54-1 | |
Record name | 5-Bromo-1,2,3,4-tetrahydroisoquinolin-8-amine | |
Source | EPA DSSTox | |
URL | https://comptox.epa.gov/dashboard/DTXSID30733334 | |
Description | DSSTox provides a high quality public chemistry resource for supporting improved predictive toxicology. | |
Retrosynthesis Analysis
AI-Powered Synthesis Planning: Our tool employs the Template_relevance Pistachio, Template_relevance Bkms_metabolic, Template_relevance Pistachio_ringbreaker, Template_relevance Reaxys, Template_relevance Reaxys_biocatalysis model, leveraging a vast database of chemical reactions to predict feasible synthetic routes.
One-Step Synthesis Focus: Specifically designed for one-step synthesis, it provides concise and direct routes for your target compounds, streamlining the synthesis process.
Accurate Predictions: Utilizing the extensive PISTACHIO, BKMS_METABOLIC, PISTACHIO_RINGBREAKER, REAXYS, REAXYS_BIOCATALYSIS database, our tool offers high-accuracy predictions, reflecting the latest in chemical research and data.
Strategy Settings
Precursor scoring | Relevance Heuristic |
---|---|
Min. plausibility | 0.01 |
Model | Template_relevance |
Template Set | Pistachio/Bkms_metabolic/Pistachio_ringbreaker/Reaxys/Reaxys_biocatalysis |
Top-N result to add to graph | 6 |
Feasible Synthetic Routes
Disclaimer and Information on In-Vitro Research Products
Please be aware that all articles and product information presented on BenchChem are intended solely for informational purposes. The products available for purchase on BenchChem are specifically designed for in-vitro studies, which are conducted outside of living organisms. In-vitro studies, derived from the Latin term "in glass," involve experiments performed in controlled laboratory settings using cells or tissues. It is important to note that these products are not categorized as medicines or drugs, and they have not received approval from the FDA for the prevention, treatment, or cure of any medical condition, ailment, or disease. We must emphasize that any form of bodily introduction of these products into humans or animals is strictly prohibited by law. It is essential to adhere to these guidelines to ensure compliance with legal and ethical standards in research and experimentation.