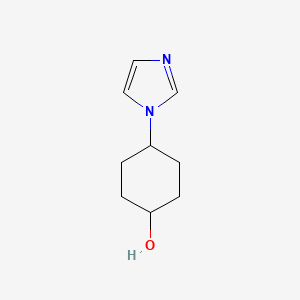
4-Imidazol-1-yl-cyclohexanol
- Click on QUICK INQUIRY to receive a quote from our team of experts.
- With the quality product at a COMPETITIVE price, you can focus more on your research.
Overview
Description
4-Imidazol-1-yl-cyclohexanol is a chemical compound that features a cyclohexanol ring substituted with an imidazole group at the 4-position. This compound is part of the broader class of imidazole derivatives, which are known for their diverse applications in various fields such as pharmaceuticals, agrochemicals, and materials science .
Preparation Methods
Synthetic Routes and Reaction Conditions
The synthesis of 4-Imidazol-1-yl-cyclohexanol typically involves the cyclization of appropriate precursors under specific conditions. One common method involves the Ullmann reaction, where p-bromoanisole and imidazole are used as starting materials. The reaction is carried out in the presence of a catalyst, alkali, and a suitable solvent. After the reaction, the product is extracted, purified, and crystallized to obtain the desired compound .
Industrial Production Methods
Industrial production of this compound may involve similar synthetic routes but on a larger scale. The process is optimized for higher yields and purity, often involving continuous flow reactors and advanced purification techniques to meet industrial standards .
Chemical Reactions Analysis
Types of Reactions
4-Imidazol-1-yl-cyclohexanol undergoes various chemical reactions, including:
Oxidation: The hydroxyl group can be oxidized to form ketones or aldehydes.
Reduction: The imidazole ring can be reduced under specific conditions.
Substitution: The imidazole ring can participate in nucleophilic substitution reactions.
Common Reagents and Conditions
Oxidation: Common oxidizing agents include potassium permanganate and chromium trioxide.
Reduction: Reducing agents such as lithium aluminum hydride can be used.
Substitution: Nucleophiles like amines or thiols can be employed in substitution reactions.
Major Products Formed
The major products formed from these reactions depend on the specific conditions and reagents used. For example, oxidation of the hydroxyl group can yield cyclohexanone derivatives, while substitution reactions can introduce various functional groups onto the imidazole ring .
Scientific Research Applications
Imidazole-Based Supramolecular Complexes
The formation of supermolecules via noncovalent bonds can influence the function of living molecules within biological systems, improving drug solubility, bioavailability, biocompatibility, drug targeting, and curative effects, while also reducing multidrug resistance, toxicity, and adverse effects . Imidazole-based supermolecules, such as I1 , I2 , I3 and I4 , are used in clinical settings and possess potential for the development of supramolecular species . Imidazole-based supermolecules have applications such as anticancer, antibacterial, antifungal, antiparasitic, antidiabetic, antihypertensive, and anti-inflammatory medicinal agents, as well as use as ion receptors, imaging agents, and pathologic probes .
Anticancer Activity
Many studies show the potential of imidazole in anticancer applications . HO-1 overexpression has been reported in several types of human malignancies, and the inhibition of HO-1 is considered a valuable anticancer approach . In fragment-based joining experiments, the benzyl group was joined to the imidazole inside the binding pocket of HO-1 . A compound (4d ) has been found to inhibit HO-1 with an IC50 of 1.03 μM . Several compounds have been developed and evaluated for antitumor potential against different cancer cell lines :
Examples of Imidazole-based Compounds with Anticancer Activity :
Compounds | IC50 value (C6) | IC50 value (HepG2) |
---|---|---|
20a | 27.0 ± 1.41 | 50.0 ± 5.0 |
20b | 20 ± 2.0 | 26.33 ± 1.53 |
20c | 32.67 ± 6.43 | 275.0 ± 35.36 |
20d | 22.0 ± 3.61 | 29.33 ± 1.15 |
20e | 16.33 ± 2.31 | 31.67 ± 7.23 |
20f | 19.50 ± 2.12 | 28.67 ± 1.15 |
20g | 15.67 ± 2.52 | 58.33 ± 2.89 |
20h | > 500 | > 500 |
20i | 24.33 ± 4.04 | > 500 |
20j | 19.33 ± 2.31 | > 500 |
Cisplatin | 23.0 ± 1.73 | 46.67 ± 7.64 |
Compounds | Cancer cells (IC50 µM) (A549) | Cancer cells (IC50 µM) (MCF-7) | Cancer cells (IC50 µM) (HEP-G2) | Cancer cells (IC50 µM) (OVCAR-3) |
---|---|---|---|---|
21a | 119.3 ± 29.9 | 13.49 ± 0.16 | 24.2 ± 0.32 | 16.91 ± 0.37 |
21b | 19.17 ± 0.43 | 18.09 ± 0.28 | 59.13 ± 0.92 | 24.7 ± 1.69 |
21c | 17.41 ± 0.16 | 16.04 ± 0.24 | 140.85 ± 0.88 | 34.44 ± 1.55 |
21d | 35.89 ± 0.84 | 32.55 ± 3.26 | 36.54 ± 1.35 | 36.48 ± 1.36 |
22a | 12.47 ± 0.18 | 12.12 ± 0.10 | 15.44 ± 0.25 | 16.09 ± 0.39 |
22b | 41.05 ± 1.61 | 53.54 ± 1.12 | 117.28 ± 2.42 | 59.01 ± 8.91 |
22c | > 314 | 254.9 ± 13.6 | > 314 | 299.52 ± 9.27 |
22d | 15.79 ± 0.49 | 13.42 ± 0.24 | 17.6 ± 0.25 | 16.13 ± 0.32 |
23a | 10.3 ± 0.13 | 9.65 ± 0.06 | 10.16 ± 0.08 | 10.5 ± 0.10 |
23b | 54.12 ± 1.20 | 53.19 ± 0.77 | 64.91 ± 0.24 | 28.71 ± 1.44 |
23c | 56.21 ± 0.96 | 56.09 ± 0.14 | 36.61 ± 1.89 | 11.4 ± 0.24 |
23d | 19.53 ± 0.71 | 14.73 ± 0.09 | 15.49 ± 0.16 | 14.04 ± 0.29 |
24a | 10.73 ± 0.58 | 9.73 ± 0.16 | 10.33 ± 0.06 | 10.34 ± 0.19 |
24b | 11.64 ± 0.25 | 11.14 ± 0.07 | 32.16 ± 1.83 | 12.55 ± 0.12 |
24c | 22.36 ± 0.54 | 21.12 ± 0.53 | 58.74 ± 0.75 | 13.29 ± 0.47 |
24d | 50.45 ± 0.82 | 54.41 ± 0.72 | 56.45 ± 0.86 | 33.13 ± 0.14 |
25a | 14.59 ± 0.40 | 10.38 ± 0.08 | 36.13 ± 0.75 | 22.44 ± 0.47 |
25b | 10.76 ± 0.29 | 10.15 ± 0.06 | 42.05 ± 0.91 | 16.32 ± 0.45 |
25c | 10.27 ± 0.15 | 11.12 ± 0.20 | 50.24 ± 0.88 | 14.88 ± 0.67 |
25d | 24.06 ± 0.08 | 22.93 ± 0.49 | 21.38 ± 0.68 | 0.14.22 ± 0.33 |
26a | 9.73 ± 0.07 | 8.91 ± 0.07 | 10.93 ± 0.10 | 10.76 ± 0.12 |
26b | 11.79 ± 0.27 | 11.34 ± 0.17 | 47.88 ± 0.76 | 13.76 ± 0.27 |
26c | 16.92 ± 0.61 | 11.93 ± 0.14 | 32.92 ± 0.38 | 13.4 ± 0.33 |
26d | 81.48 ± 1.40 | 35.69 ± 0.47 | 95.7 ± 2.44 | 42.24 ± 2.43 |
DOX | 0.46 ± 0.01 | 0.42 ± 0.01 | 0.72 ± 0.01 | 3.95 ± 0.09 |
Cisplatin | 7.31 ± 0.44 | 11.7 ± 0.12 | 3.97 ± 0.04 | 16.04 ± 0.74 |
Antibacterial Activity
Some derivatives of 1,3-diazole exhibit antibacterial activities . For example, compounds 1a and 1b showed good antimicrobial potential against S. aureus, E. coli, and B. subtilis . Compound 4 h shows the most potent activity against Staphylococcus aureus, Bacillus subtilis, Escherichia coli, and Pseudomonas aeruginosa .
Other Activities
Imidazole compounds have demonstrated a range of activities, leading to their use in treatments for various conditions :
Mechanism of Action
The mechanism of action of 4-Imidazol-1-yl-cyclohexanol involves its interaction with specific molecular targets. The imidazole ring can interact with enzymes and receptors, modulating their activity. This interaction can lead to various biological effects, such as inhibition of microbial growth or modulation of metabolic pathways .
Comparison with Similar Compounds
Similar Compounds
- 4-(Imidazol-1-yl)phenol
- 4-(Imidazol-1-yl)acetophenone
- 4-(Imidazol-1-yl)benzoic acid
Uniqueness
4-Imidazol-1-yl-cyclohexanol is unique due to its specific structural features, which confer distinct chemical and biological properties.
Properties
Molecular Formula |
C9H14N2O |
---|---|
Molecular Weight |
166.22 g/mol |
IUPAC Name |
4-imidazol-1-ylcyclohexan-1-ol |
InChI |
InChI=1S/C9H14N2O/c12-9-3-1-8(2-4-9)11-6-5-10-7-11/h5-9,12H,1-4H2 |
InChI Key |
BFRIPECZNDHAOZ-UHFFFAOYSA-N |
Canonical SMILES |
C1CC(CCC1N2C=CN=C2)O |
Origin of Product |
United States |
Disclaimer and Information on In-Vitro Research Products
Please be aware that all articles and product information presented on BenchChem are intended solely for informational purposes. The products available for purchase on BenchChem are specifically designed for in-vitro studies, which are conducted outside of living organisms. In-vitro studies, derived from the Latin term "in glass," involve experiments performed in controlled laboratory settings using cells or tissues. It is important to note that these products are not categorized as medicines or drugs, and they have not received approval from the FDA for the prevention, treatment, or cure of any medical condition, ailment, or disease. We must emphasize that any form of bodily introduction of these products into humans or animals is strictly prohibited by law. It is essential to adhere to these guidelines to ensure compliance with legal and ethical standards in research and experimentation.