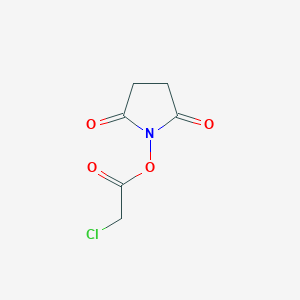
N-(Chloroacetoxy)succinimide
Overview
Description
N-(Chloroacetoxy)succinimide is an organochlorine compound used in the preparation of organometallic complexes and other synthetic probes for the labeling of proteins . It acts as an electrophilic reagent for the synthesis of peptides and amines .
Synthesis Analysis
N-(Chloroacetoxy)succinimide can be synthesized through various methods. One such method involves the use of N-chlorosuccinimide (NCS) as a versatile reagent . NCS mediates or catalyzes many chemical reactions, including halocyclizations, formation of heterocyclic systems, formation of new carbon-carbon bonds, rearrangements, and functional group transformations .Molecular Structure Analysis
The molecular formula of N-(Chloroacetoxy)succinimide is C6H6ClNO4 . Its molecular weight is 191.57 g/mol . The InChIKey of the compound is OWZGNRBFMASABS-UHFFFAOYSA-N .Chemical Reactions Analysis
N-(Chloroacetoxy)succinimide is involved in various chemical reactions. For instance, it can be used as a source for chlorine in radical reactions and various electrophilic additions . It can also be used in the chlorination of thiols .Physical And Chemical Properties Analysis
N-(Chloroacetoxy)succinimide has a molecular weight of 191.57 g/mol . It has a hydrogen bond donor count of 0 and a hydrogen bond acceptor count of 4 . The compound has a rotatable bond count of 3 .Scientific Research Applications
Synthesis of Peptides and Amines
N-(Chloroacetoxy)succinimide: is utilized as an electrophilic reagent in the synthesis of peptides and amines . Its reactivity allows for the introduction of acetoxy groups into these molecules, which can be pivotal for the formation of peptide bonds and the modification of amino groups.
Labeling of Proteins
This compound is used in the preparation of synthetic probes for the labeling of proteins . It can react with nucleophilic sites on proteins, enabling researchers to tag proteins with various markers for identification and tracking in biological systems.
Preparation of Organometallic Complexes
Researchers employ N-(Chloroacetoxy)succinimide in the creation of organometallic complexes . These complexes are often used in catalysis and materials science, and the introduction of the chloroacetoxy moiety can influence the properties and reactivity of these complexes.
Mitigation of Succinimide Accumulation
In the pharmaceutical industry, particularly in the development of monoclonal antibodies, controlling succinimide formation is crucial . N-(Chloroacetoxy)succinimide may play a role in the mitigation of succinimide accumulation, which can affect the potency and stability of therapeutic proteins.
Modification of Aspartic Acid Residues
The compound is involved in the study of modifications at aspartic acid residues within proteins . Understanding these modifications can lead to insights into protein stability and function, which is significant for the design of stable and effective biopharmaceuticals.
Formulation Studies
N-(Chloroacetoxy)succinimide: can be used in formulation studies to explore its effects on the stability and efficacy of therapeutic molecules . By examining how this compound interacts with other components in a formulation, researchers can optimize the storage and handling conditions of pharmaceuticals.
Electrophilic Reactions
Due to its electrophilic nature, N-(Chloroacetoxy)succinimide is a valuable reagent for various electrophilic reactions in organic synthesis . It can be used to introduce functional groups into organic molecules, facilitating the synthesis of a wide range of chemical compounds.
Chemical Research and Material Science
Lastly, N-(Chloroacetoxy)succinimide finds applications in broader chemical research and material science . Its unique reactivity profile makes it a versatile tool for the synthesis and modification of materials with specific desired properties.
Mechanism of Action
Target of Action
N-(Chloroacetoxy)succinimide (NCAS) is an organochlorine compound primarily used in the preparation of organometallic complexes and other synthetic probes . Its primary targets are proteins, peptides, and amines . It acts as an electrophilic reagent, which means it has a tendency to attract electrons and react with nucleophilic (electron-rich) targets .
Mode of Action
The mode of action of NCAS involves its interaction with its targets through electrophilic substitution reactions . As an electrophilic reagent, NCAS can form covalent bonds with nucleophilic sites on proteins, peptides, and amines . This interaction results in the modification of these targets, which can lead to changes in their structure and function .
Biochemical Pathways
Given its role as an electrophilic reagent, it can be inferred that ncas may affect various biochemical pathways involving proteins, peptides, and amines . The downstream effects of these interactions would depend on the specific targets and the nature of the modifications induced by NCAS .
Pharmacokinetics
These properties would play a crucial role in determining the bioavailability of ncas and its ultimate effectiveness as a reagent .
Result of Action
The result of NCAS’s action is the modification of its targets, which can lead to changes in their structure and function . These changes can have various molecular and cellular effects, depending on the specific targets and the nature of the modifications .
Action Environment
The action, efficacy, and stability of NCAS can be influenced by various environmental factors. These may include factors such as temperature, pH, and the presence of other chemicals or reagents .
Future Directions
While specific future directions for N-(Chloroacetoxy)succinimide were not found in the search results, the compound’s use in the preparation of organometallic complexes and other synthetic probes for the labeling of proteins suggests potential applications in biochemical research and drug development .
properties
IUPAC Name |
(2,5-dioxopyrrolidin-1-yl) 2-chloroacetate | |
---|---|---|
Source | PubChem | |
URL | https://pubchem.ncbi.nlm.nih.gov | |
Description | Data deposited in or computed by PubChem | |
InChI |
InChI=1S/C6H6ClNO4/c7-3-6(11)12-8-4(9)1-2-5(8)10/h1-3H2 | |
Source | PubChem | |
URL | https://pubchem.ncbi.nlm.nih.gov | |
Description | Data deposited in or computed by PubChem | |
InChI Key |
OWZGNRBFMASABS-UHFFFAOYSA-N | |
Source | PubChem | |
URL | https://pubchem.ncbi.nlm.nih.gov | |
Description | Data deposited in or computed by PubChem | |
Canonical SMILES |
C1CC(=O)N(C1=O)OC(=O)CCl | |
Source | PubChem | |
URL | https://pubchem.ncbi.nlm.nih.gov | |
Description | Data deposited in or computed by PubChem | |
Molecular Formula |
C6H6ClNO4 | |
Source | PubChem | |
URL | https://pubchem.ncbi.nlm.nih.gov | |
Description | Data deposited in or computed by PubChem | |
DSSTOX Substance ID |
DTXSID80501641 | |
Record name | 1-[(Chloroacetyl)oxy]pyrrolidine-2,5-dione | |
Source | EPA DSSTox | |
URL | https://comptox.epa.gov/dashboard/DTXSID80501641 | |
Description | DSSTox provides a high quality public chemistry resource for supporting improved predictive toxicology. | |
Molecular Weight |
191.57 g/mol | |
Source | PubChem | |
URL | https://pubchem.ncbi.nlm.nih.gov | |
Description | Data deposited in or computed by PubChem | |
Product Name |
N-(Chloroacetoxy)succinimide | |
CAS RN |
27243-15-8 | |
Record name | 1-[(Chloroacetyl)oxy]pyrrolidine-2,5-dione | |
Source | EPA DSSTox | |
URL | https://comptox.epa.gov/dashboard/DTXSID80501641 | |
Description | DSSTox provides a high quality public chemistry resource for supporting improved predictive toxicology. | |
Retrosynthesis Analysis
AI-Powered Synthesis Planning: Our tool employs the Template_relevance Pistachio, Template_relevance Bkms_metabolic, Template_relevance Pistachio_ringbreaker, Template_relevance Reaxys, Template_relevance Reaxys_biocatalysis model, leveraging a vast database of chemical reactions to predict feasible synthetic routes.
One-Step Synthesis Focus: Specifically designed for one-step synthesis, it provides concise and direct routes for your target compounds, streamlining the synthesis process.
Accurate Predictions: Utilizing the extensive PISTACHIO, BKMS_METABOLIC, PISTACHIO_RINGBREAKER, REAXYS, REAXYS_BIOCATALYSIS database, our tool offers high-accuracy predictions, reflecting the latest in chemical research and data.
Strategy Settings
Precursor scoring | Relevance Heuristic |
---|---|
Min. plausibility | 0.01 |
Model | Template_relevance |
Template Set | Pistachio/Bkms_metabolic/Pistachio_ringbreaker/Reaxys/Reaxys_biocatalysis |
Top-N result to add to graph | 6 |
Feasible Synthetic Routes
Disclaimer and Information on In-Vitro Research Products
Please be aware that all articles and product information presented on BenchChem are intended solely for informational purposes. The products available for purchase on BenchChem are specifically designed for in-vitro studies, which are conducted outside of living organisms. In-vitro studies, derived from the Latin term "in glass," involve experiments performed in controlled laboratory settings using cells or tissues. It is important to note that these products are not categorized as medicines or drugs, and they have not received approval from the FDA for the prevention, treatment, or cure of any medical condition, ailment, or disease. We must emphasize that any form of bodily introduction of these products into humans or animals is strictly prohibited by law. It is essential to adhere to these guidelines to ensure compliance with legal and ethical standards in research and experimentation.