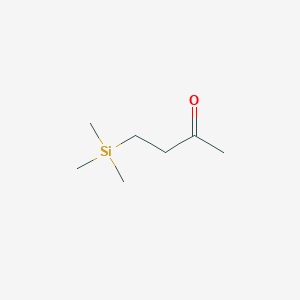
4-Trimethylsilyl-2-butanone
- Click on QUICK INQUIRY to receive a quote from our team of experts.
- With the quality product at a COMPETITIVE price, you can focus more on your research.
Overview
Description
4-(Trimethylsilyl)-2-butanone is an organosilicon compound characterized by the presence of a trimethylsilyl group attached to a butanone backbone. This compound is notable for its utility in organic synthesis, particularly as a reagent and intermediate in various chemical reactions. The trimethylsilyl group imparts unique properties to the molecule, such as increased volatility and chemical stability, making it valuable in both laboratory and industrial settings.
Preparation Methods
Synthetic Routes and Reaction Conditions
The synthesis of 4-(Trimethylsilyl)-2-butanone typically involves the reaction of 2-butanone with a trimethylsilylating agent. One common method is the reaction of 2-butanone with trimethylsilyl chloride in the presence of a base such as triethylamine. The reaction proceeds under mild conditions, often at room temperature, and yields the desired product with high efficiency .
Industrial Production Methods
On an industrial scale, the production of 4-(Trimethylsilyl)-2-butanone can be achieved through a similar process, but with optimizations for large-scale synthesis. This includes the use of continuous flow reactors and automated systems to ensure consistent quality and yield. The reaction conditions are carefully controlled to minimize by-products and maximize the efficiency of the process .
Chemical Reactions Analysis
Types of Reactions
4-(Trimethylsilyl)-2-butanone undergoes various types of chemical reactions, including:
Oxidation: The compound can be oxidized to form corresponding ketones or carboxylic acids.
Reduction: It can be reduced to form alcohols or other reduced derivatives.
Substitution: The trimethylsilyl group can be substituted with other functional groups through nucleophilic substitution reactions.
Common Reagents and Conditions
Oxidation: Common oxidizing agents include potassium permanganate and chromium trioxide.
Reduction: Reducing agents such as lithium aluminum hydride and sodium borohydride are frequently used.
Substitution: Reagents like halides and nucleophiles are used under basic or acidic conditions to facilitate substitution reactions.
Major Products Formed
Oxidation: Ketones and carboxylic acids.
Reduction: Alcohols.
Substitution: Various substituted derivatives depending on the nucleophile used.
Scientific Research Applications
The search results provide information on compounds structurally similar to "4-(Trimethylsilyl)-2-butanone". However, none of the search results directly discuss the applications of "4-(Trimethylsilyl)-2-butanone". Instead, the search results discuss "2-Butanone" , "3-Methyl-2-[(trimethylsilyl)oxy]butanenitrile", "Polyphenol-Containing Nanoparticles" , and "4-(Trimethylsilyl)-3-butyn-2-one" .
2-Butanone (Methyl Ethyl Ketone or MEK)
2-Butanone, also known as methyl ethyl ketone (MEK), serves as a solvent in various industrial applications. These applications include:
- Paints and lacquers
- Rubber cement
- Printing inks
- Paint removers
- Vinyl films
- Resins and rosins
- Polystyrene
- Chlorinated rubber
- Polyurethane
- Acrylic coatings
- Cleaning solutions
- Production of synthetic leathers, transparent paper, and aluminum foil
- Degreasing of metals
- Extraction solvent
- Dewaxing of lubricating oils
- Solvent for smokeless powders
3-Methyl-2-[(trimethylsilyl)oxy]butanenitrile
Study | Focus | Key Findings |
---|---|---|
Study A | Antimicrobial | Demonstrated significant antibacterial activity against Staphylococcus aureus with MIC values comparable to established antibiotics. |
Study B | Cytotoxicity | Reported IC50 values indicating moderate cytotoxicity against Hela cells, suggesting potential for cancer therapy applications. |
Study C | Mechanistic Insights | Proposed that the compound interacts with cellular signaling pathways, leading to altered gene expression related to stress responses. |
Mechanism of Action
The mechanism of action of 4-(Trimethylsilyl)-2-butanone involves the reactivity of the trimethylsilyl group. This group can act as a protecting group for hydroxyl and other reactive functional groups, preventing unwanted side reactions during synthesis. The trimethylsilyl group can be selectively removed under mild conditions, revealing the protected functional group for further reactions .
Comparison with Similar Compounds
Similar Compounds
- Trimethylsilyl chloride
- Trimethylsilyl fluoride
- Trimethylsilyl bromide
- Trimethylsilyl iodide
- Trimethylsilyl cyanide
- Trimethylsilyl azide
- Trimethylsilyl trifluoromethanesulfonate
Uniqueness
4-(Trimethylsilyl)-2-butanone is unique due to its specific structure, which combines the reactivity of the trimethylsilyl group with the functional versatility of the butanone backbone. This combination allows for a wide range of chemical transformations and applications, making it a valuable compound in both research and industrial contexts .
Properties
CAS No. |
13506-88-2 |
---|---|
Molecular Formula |
C7H16OSi |
Molecular Weight |
144.29 g/mol |
IUPAC Name |
4-trimethylsilylbutan-2-one |
InChI |
InChI=1S/C7H16OSi/c1-7(8)5-6-9(2,3)4/h5-6H2,1-4H3 |
InChI Key |
YGRIAGFGPDBPCM-UHFFFAOYSA-N |
Canonical SMILES |
CC(=O)CC[Si](C)(C)C |
Origin of Product |
United States |
Disclaimer and Information on In-Vitro Research Products
Please be aware that all articles and product information presented on BenchChem are intended solely for informational purposes. The products available for purchase on BenchChem are specifically designed for in-vitro studies, which are conducted outside of living organisms. In-vitro studies, derived from the Latin term "in glass," involve experiments performed in controlled laboratory settings using cells or tissues. It is important to note that these products are not categorized as medicines or drugs, and they have not received approval from the FDA for the prevention, treatment, or cure of any medical condition, ailment, or disease. We must emphasize that any form of bodily introduction of these products into humans or animals is strictly prohibited by law. It is essential to adhere to these guidelines to ensure compliance with legal and ethical standards in research and experimentation.