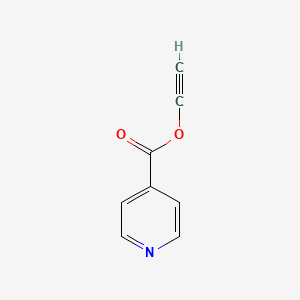
Ethynyl pyridine-4-carboxylate
- Click on QUICK INQUIRY to receive a quote from our team of experts.
- With the quality product at a COMPETITIVE price, you can focus more on your research.
Overview
Description
Ethynyl pyridine-4-carboxylate is a pyridine derivative featuring an ethynyl group (-C≡CH) and a carboxylate ester (-COOR) at the 4-position of the pyridine ring. For instance, palladium-catalyzed decarboxylative coupling reactions (as seen in alkynyl carboxylic acids and aryl tosylates) are likely applicable for its synthesis, given the prevalence of such methods in forming ethynyl-substituted pyridines . The ethynyl group enhances reactivity for further functionalization, while the carboxylate ester improves solubility and serves as a protecting group for carboxylic acids.
Preparation Methods
Synthetic Routes and Reaction Conditions: The synthesis of ethynyl pyridine-4-carboxylate typically involves the reaction of pyridine-4-carboxylic acid with ethynylating agents under specific conditions. One common method is the Sonogashira coupling reaction, which involves the use of palladium catalysts and copper co-catalysts in the presence of a base. The reaction is carried out in an inert atmosphere, usually under nitrogen or argon, to prevent oxidation.
Industrial Production Methods: Industrial production of this compound follows similar synthetic routes but on a larger scale. The process involves optimizing reaction conditions to maximize yield and minimize by-products. Continuous flow reactors and automated systems are often employed to ensure consistent quality and efficiency.
Chemical Reactions Analysis
Types of Reactions: Ethynyl pyridine-4-carboxylate undergoes various chemical reactions, including:
Oxidation: The ethynyl group can be oxidized to form corresponding carbonyl compounds.
Reduction: The compound can be reduced to form ethyl or vinyl derivatives.
Substitution: The ethynyl group can participate in nucleophilic substitution reactions, leading to the formation of various substituted derivatives.
Common Reagents and Conditions:
Oxidation: Common oxidizing agents include potassium permanganate and chromium trioxide.
Reduction: Reducing agents such as lithium aluminum hydride and sodium borohydride are used.
Substitution: Nucleophiles like amines, thiols, and halides are employed under basic or acidic conditions.
Major Products: The major products formed from these reactions include carbonyl compounds, ethyl or vinyl derivatives, and various substituted pyridine derivatives.
Scientific Research Applications
Ethynyl pyridine-4-carboxylate has a wide range of applications in scientific research:
Chemistry: It is used as a building block in the synthesis of complex organic molecules and heterocyclic compounds.
Biology: The compound is studied for its potential biological activities, including antimicrobial and anticancer properties.
Medicine: Research is ongoing to explore its potential as a pharmaceutical intermediate and its role in drug development.
Industry: It is used in the production of advanced materials, including polymers and catalysts.
Mechanism of Action
The mechanism of action of ethynyl pyridine-4-carboxylate involves its interaction with specific molecular targets and pathways. The ethynyl group can participate in π-π interactions and hydrogen bonding, influencing the compound’s binding affinity and selectivity. The carboxylate group can form ionic interactions with target molecules, enhancing its biological activity.
Comparison with Similar Compounds
Structural and Functional Group Variations
Ethynyl vs. Chloro Substituents
- Ethynyl Pyridine-4-Carboxylate: The ethynyl group enables conjugation and participation in click chemistry or Sonogashira coupling.
- (E)-Methyl 3-(4-Chloropyridin-3-yl)acrylate (): The chloro substituent increases electrophilicity but reduces opportunities for cross-coupling reactions compared to ethynyl groups.
Carboxylate Esters
- Ethyl-4-Phenyl Pyrimidine-5-Carboxylate (): Pyrimidine rings (two nitrogen atoms) confer distinct electronic effects compared to pyridine (one nitrogen), altering solubility and hydrogen-bonding capacity.
Physicochemical Properties
Melting Points and Solubility
- Pyridine derivatives with bulky substituents (e.g., 2-Amino-4-(2-Chloro-5-(4-Substituted Phenyl)Pyridin-3-Yl)-1-(4-Substituted Phenyl)Pyridine in ) exhibit higher melting points (268–287°C) due to intermolecular interactions. This compound likely has a lower melting point, assuming reduced symmetry compared to halogenated analogs.
- Carboxylate esters generally enhance solubility in organic solvents. For example, Ethyl 2-Allylsulfanyl-4-(4-Methoxyphenyl)-1,4-Dihydropyrimidine-5-Carboxylate () shows moderate solubility in dichloromethane, a trend expected for this compound.
Spectroscopic Data
- NMR Shifts : Pyridine-4-carboxylate derivatives coordinated to Fe(CN)₅ () show distinct proton shifts (δ 9.03 ppm for aromatic protons), which may differ in ethynyl analogs due to electron-withdrawing effects of the ethynyl group.
- IR Spectra : Stretching frequencies for ester carbonyl groups (~1700 cm⁻¹) and ethynyl C≡C (~2100 cm⁻¹) would dominate, as seen in related alkynyl compounds .
Catalytic Coupling Reactions
- This compound is amenable to palladium-catalyzed reactions, similar to 3-(m-Tolylethynyl)Pyridine (3ch, 82% yield in ). The carboxylate ester may stabilize intermediates, improving reaction efficiency.
- In contrast, chloro-substituted pyridines () require harsher conditions (e.g., CuI catalysts) for cross-coupling.
Coordination Chemistry
- Pyridine-4-carboxylate forms coordination complexes with metals like iron (), but ethynyl substituents may destabilize such complexes due to steric or electronic effects.
Data Tables
Table 1: Key Properties of this compound and Analogs
*Hypothetical data inferred from analogs.
Table 2: Reaction Yields and Conditions
Biological Activity
Ethynyl pyridine-4-carboxylate is a compound of interest in medicinal chemistry due to its potential biological activities. This article explores its biological activity, including anticancer properties, mechanisms of action, and related research findings.
Chemical Structure and Properties
This compound features a pyridine ring substituted with an ethynyl group and a carboxylate moiety. This structure is significant because it allows for interactions with various biological targets, particularly in cancer therapy.
Biological Activity Overview
-
Anticancer Activity
- Ethynyl pyridine derivatives have shown efficacy against various cancer cell lines. For instance, studies indicate that compounds with similar structures exhibit cytotoxic effects against HepG2 (liver), Caco2 (colon), and MCF-7 (breast) cancer cells .
- The mechanism often involves the inhibition of key signaling pathways associated with tumor growth and metastasis.
-
Mechanisms of Action
- FGFR Inhibition : Some derivatives, such as 1H-pyrrolo[2,3-b]pyridine, demonstrate potent inhibition of fibroblast growth factor receptors (FGFRs), which are crucial in tumor proliferation and survival .
- Targeting Enzymatic Pathways : Pyridine derivatives can act on carbonic anhydrase, which plays a role in various physiological processes including acid-base balance and respiration, making them potential candidates for targeting metabolic pathways in cancer .
Table 1: Summary of Biological Activities
Compound | Target/Activity | IC50 Value (µM) | Cell Line Tested |
---|---|---|---|
This compound | FGFR Inhibition | 7-712 | 4T1 (breast cancer) |
Compound 4 | Cytotoxicity against HepG2 | Not specified | HepG2 |
Compound 8 | Cytotoxicity against MCF-7 | Moderate | MCF-7 |
Study Insights
- A study highlighted that compound 4 exhibited strong targeting ability to sarcoma cells in vivo, suggesting its potential as an anticancer agent . The cytopathological examination confirmed the effectiveness of these compounds in inhibiting tumor growth.
- Another research focused on the synthesis and evaluation of pyridine derivatives indicated their diverse biological activities including anti-inflammatory and antimicrobial properties, emphasizing their therapeutic potential beyond oncology .
Q & A
Basic Research Questions
Q. What are the standard synthetic routes for ethynyl pyridine-4-carboxylate, and how can reaction conditions be optimized?
this compound is typically synthesized via Sonogashira coupling between a halogenated pyridine-4-carboxylate precursor and a terminal alkyne. Optimization involves tuning catalyst systems (e.g., Pd(PPh₃)₂Cl₂/CuI), solvent polarity (e.g., THF vs. DMF), and base selection (e.g., triethylamine or K₂CO₃) to improve yield and reduce side reactions like alkyne dimerization . Reaction monitoring via TLC or HPLC-MS is critical for identifying intermediates and endpoint determination.
Q. How can spectroscopic techniques (NMR, IR, MS) confirm the structural integrity of this compound?
- ¹H/¹³C NMR : The ethynyl proton appears as a singlet near δ 2.5–3.5 ppm, while the pyridine ring protons show distinct splitting patterns (e.g., para-substitution at C4).
- IR : A sharp peak ~2100–2260 cm⁻¹ confirms the C≡C stretch.
- HRMS : Exact mass matching (e.g., [M+H]⁺ calculated for C₉H₇NO₂: 161.0477) ensures molecular formula consistency . Cross-validation with X-ray crystallography (if crystalline) provides definitive confirmation of spatial arrangement .
Q. What are the stability considerations for this compound under varying storage conditions?
The compound is sensitive to moisture and light due to the reactive ethynyl group. Stability studies recommend storage in anhydrous DMSO or under argon at −20°C. Accelerated degradation studies (e.g., 40°C/75% RH for 14 days) coupled with HPLC analysis quantify decomposition products like oxidized alkynes or ester hydrolysis derivatives .
Advanced Research Questions
Q. How can computational methods (DFT, MD) predict the reactivity of this compound in cross-coupling reactions?
Density Functional Theory (DFT) calculations model transition states to identify energy barriers for Pd-catalyzed coupling steps. Molecular dynamics (MD) simulations assess solvent effects on reaction kinetics. For example, solvation-free energy calculations in polar aprotic solvents correlate with experimental yield trends .
Q. What strategies resolve contradictions in catalytic activity data between this compound derivatives?
Discrepancies in catalytic performance (e.g., Suzuki-Miyaura coupling yields) may arise from steric effects or electronic modulation. Systematic studies using Hammett plots (σpara values for substituents) or XPS analysis of catalyst surfaces can isolate contributing factors. Contradictory data should be analyzed via multivariate regression to deconvolute variables .
Q. How does the electronic nature of the pyridine ring influence the compound’s application in metal-organic frameworks (MOFs)?
Pyridine’s Lewis basicity enables coordination with metal nodes (e.g., Zn²⁺, Cu²⁺) in MOF synthesis. Frontier molecular orbital (FMO) analysis predicts charge-transfer interactions. Experimental validation via BET surface area measurements and gas adsorption isotherms quantifies framework porosity enhancements compared to non-functionalized analogs .
Q. What mechanistic insights explain this compound’s role in photoactive drug delivery systems?
Photoisomerization or radical generation under UV light can be studied using time-resolved ESR or transient absorption spectroscopy. For instance, the ethynyl group may act as a photosensitizer, generating singlet oxygen (¹O₂) for targeted cytotoxicity. In vitro assays (e.g., MTT on cancer cell lines) validate efficacy, while LC-MS tracks metabolite formation .
Q. Methodological Guidance
Designing a structure-activity relationship (SAR) study for this compound derivatives
- Variable selection : Modify substituents at the pyridine C3/C5 positions or ester groups.
- Assays : Measure IC₅₀ in enzyme inhibition (e.g., kinase assays) or cellular uptake via fluorescence tagging.
- Data analysis : Use principal component analysis (PCA) to cluster bioactivity profiles and identify critical substituents .
Addressing reproducibility challenges in scaled-up synthesis
Batch-to-batch variability often stems from inhomogeneous catalyst dispersion or oxygen contamination. Implement inline PAT (Process Analytical Technology) tools like ReactIR for real-time monitoring. Statistical DOE (Design of Experiments) models optimize parameters (e.g., stirring rate, temperature gradient) for consistent output .
Q. Data Presentation and Reporting
Best practices for reporting crystallographic data (e.g., CIF files)
Include full refinement parameters (R-factors, residual density) and validate using checkCIF/PLATON. Annotate thermal ellipsoid plots to highlight key bonding interactions (e.g., C≡C···π stacking) .
Handling conflicting spectroscopic data in publications
Disclose raw data (e.g., NMR FIDs) in supplementary materials and apply Bayesian statistics to assess confidence intervals. Cross-reference with analogous compounds in the Cambridge Structural Database (CSD) .
Properties
CAS No. |
342018-56-8 |
---|---|
Molecular Formula |
C8H5NO2 |
Molecular Weight |
147.13 g/mol |
IUPAC Name |
ethynyl pyridine-4-carboxylate |
InChI |
InChI=1S/C8H5NO2/c1-2-11-8(10)7-3-5-9-6-4-7/h1,3-6H |
InChI Key |
YPKNODVFTAQQBC-UHFFFAOYSA-N |
Canonical SMILES |
C#COC(=O)C1=CC=NC=C1 |
Origin of Product |
United States |
Disclaimer and Information on In-Vitro Research Products
Please be aware that all articles and product information presented on BenchChem are intended solely for informational purposes. The products available for purchase on BenchChem are specifically designed for in-vitro studies, which are conducted outside of living organisms. In-vitro studies, derived from the Latin term "in glass," involve experiments performed in controlled laboratory settings using cells or tissues. It is important to note that these products are not categorized as medicines or drugs, and they have not received approval from the FDA for the prevention, treatment, or cure of any medical condition, ailment, or disease. We must emphasize that any form of bodily introduction of these products into humans or animals is strictly prohibited by law. It is essential to adhere to these guidelines to ensure compliance with legal and ethical standards in research and experimentation.