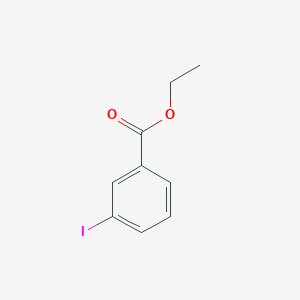
Ethyl 3-iodobenzoate
Overview
Description
Ethyl 3-iodobenzoate (C9H9IO2, CAS 58313-23-8) is an aromatic ester featuring an iodine substituent at the meta position of the benzene ring. It is synthesized via esterification of 3-iodobenzoic acid with ethanol in the presence of HCl . Key physicochemical properties include a molecular weight of 276.07 g/mol, density of 1.64 g/mL (25°C), and boiling point of 161°C at 38 mmHg . Its logP value of 3.84 indicates moderate lipophilicity , and it is sparingly soluble in water.
The compound is widely used in organic synthesis, particularly in cross-coupling reactions (e.g., Suzuki-Miyaura) to generate biphenyl derivatives , and as a precursor for radiopharmaceuticals like succinimidyl 3-iodobenzoate (SIB) for antibody labeling . Its iodine atom serves as a versatile handle for further functionalization while remaining inert under certain transition metal-catalyzed conditions .
Preparation Methods
Synthetic Routes and Reaction Conditions: Ethyl 3-iodobenzoate can be synthesized through several methods. One common approach involves the iodination of ethyl benzoate. This process typically uses iodine and a suitable oxidizing agent, such as nitric acid or hydrogen peroxide, to introduce the iodine atom into the benzene ring. The reaction is carried out under controlled conditions to ensure the selective formation of the 3-iodo derivative .
Industrial Production Methods: In an industrial setting, the production of this compound may involve the use of continuous flow reactors to enhance reaction efficiency and yield. The process parameters, such as temperature, pressure, and reactant concentrations, are optimized to achieve high purity and consistent quality of the final product .
Chemical Reactions Analysis
Types of Reactions: Ethyl 3-iodobenzoate undergoes various chemical reactions, including:
Substitution Reactions: The iodine atom in this compound can be replaced by other nucleophiles, such as amines, thiols, or alkoxides, to form a wide range of substituted benzoates.
Coupling Reactions: It can participate in palladium-catalyzed cross-coupling reactions, such as the Suzuki-Miyaura coupling, to form biaryl compounds.
Reduction Reactions: The iodine atom can be reduced to a hydrogen atom using reducing agents like sodium borohydride or lithium aluminum hydride.
Common Reagents and Conditions:
Substitution Reactions: Typical reagents include nucleophiles like sodium azide, potassium thiolate, or sodium alkoxide.
Coupling Reactions: Palladium catalysts, such as palladium acetate or palladium chloride, are used along with bases like potassium carbonate or cesium carbonate.
Reduction Reactions: Reducing agents like sodium borohydride or lithium aluminum hydride are used in solvents like ethanol or tetrahydrofuran at low temperatures.
Major Products Formed:
Substitution Reactions: Substituted benzoates with various functional groups, such as amines, thiols, or ethers.
Coupling Reactions: Biaryl compounds with diverse substituents on the aromatic rings.
Reduction Reactions: Ethyl benzoate or other reduced derivatives.
Scientific Research Applications
Chemical Properties and Structure
- Molecular Formula : CHIO
- Molecular Weight : 276.07 g/mol
- CAS Number : 58313-23-8
- Functional Groups : Iodo and ester groups
- Physical Properties :
- Density: 1.64 g/mL at 25 °C
- Boiling Point: 272 °C
Synthesis Applications
Ethyl 3-iodobenzoate is primarily used as a precursor in various synthetic pathways:
- Arylzinc Compounds : It can be converted into arylzinc bromide through reactions with organometallic reagents, facilitating the formation of carbon-carbon bonds in organic synthesis .
- Functionalized Arylmagnesium Compounds : The compound can also react with Grignard reagents to yield functionalized arylmagnesium compounds, which are useful in further synthetic applications .
- Complex Organic Molecules : this compound serves as a building block for synthesizing pharmaceuticals, agrochemicals, and materials science products. For instance, it can react with hydrazine to produce hydrazones that may be cyclized into oxadiazolines .
Biological Applications
In biological research, this compound has been identified as a precursor for synthesizing various biologically active compounds:
- Enzyme Inhibitors : It plays a role in developing enzyme inhibitors that can modulate biochemical pathways, making it valuable in drug discovery.
- Diagnostic Agents : The compound is employed in the formulation of diagnostic agents due to its ability to interact with biological systems selectively .
- Cellular Effects : Studies indicate that this compound influences cellular processes such as signaling pathways and gene expression, impacting respiratory system functions.
Industrial Applications
This compound is used in several industrial applications:
- Specialty Chemicals Production : It serves as an intermediate in producing specialty chemicals utilized across various industries, including polymers and coatings .
- Agrochemical Synthesis : The compound is involved in synthesizing agrochemicals that enhance agricultural productivity while minimizing environmental impact.
Case Study 1: Synthesis of Aryl Compounds
A study demonstrated the utility of this compound in synthesizing functionalized aryl compounds through cross-coupling reactions. The resulting arylzinc bromide was employed to create a library of aryl-substituted products with potential pharmaceutical applications.
Research has shown that derivatives of this compound exhibit significant biological activity against specific enzymes involved in metabolic pathways. This highlights its potential as a lead compound in drug development aimed at treating metabolic disorders.
Mechanism of Action
The mechanism of action of ethyl 3-iodobenzoate primarily involves its reactivity as an electrophile due to the presence of the iodine atom. This electrophilic nature allows it to participate in various substitution and coupling reactions, forming new carbon-carbon and carbon-heteroatom bonds . The molecular targets and pathways involved depend on the specific reactions and applications in which it is used.
Comparison with Similar Compounds
Halo-Substituted Benzoates
Ethyl 3-iodobenzoate is compared with chloro- and bromo-substituted analogs (Table 1).
Key Findings :
- Enzyme Interactions : In Desulfomonile tiedjei cell extracts, 3-iodobenzoate competitively inhibits dehalogenation of 3-chloro- and 3,5-dichlorobenzoates, suggesting higher binding affinity to the enzyme active site . However, its larger atomic radius (vs. Cl, Br) renders it inactive in benzoate dioxygenase due to steric hindrance .
Substituted Benzoate Esters
Comparison with methyl, amino, and methoxy derivatives (Table 2).
Key Findings :
- Synthetic Utility : The iodine atom in this compound enables regioselective iridium-catalyzed C–H borylation without oxidative addition, a contrast to bromo- or chloroarenes .
- Biological Applications : Derivatives like SIB and SGMIB are critical in targeted radionuclide therapy, leveraging the iodine atom for stable conjugation .
Physicochemical and Toxicological Profiles
This compound exhibits higher density and molecular weight compared to non-halogenated esters (e.g., ethyl benzoate: 1.05 g/mL, 150.17 g/mol). Its iodine atom contributes to greater lipophilicity (logP 3.84 vs. 2.3 for ethyl benzoate) . Safety data classify it as a skin/eye irritant , whereas chloro- and bromo-analogs show similar hazards but differ in metabolic pathways due to halogen-specific interactions.
Biological Activity
Ethyl 3-iodobenzoate is an organic compound with significant biological activity, particularly in the fields of medicinal chemistry and pharmacology. This article explores its biological properties, mechanisms of action, and potential applications based on diverse research findings.
Chemical Structure : this compound has the molecular formula C9H9IO2 and features an ethyl ester group attached to a benzoate with an iodine atom at the meta position. Its structure can be represented as follows:
Mechanisms of Biological Activity
- Antimicrobial Properties : this compound exhibits antimicrobial activity against various pathogens. Studies suggest that halogenated compounds, including iodinated benzoates, can disrupt microbial cell membranes and inhibit growth by interfering with metabolic processes.
- Anticancer Potential : Research indicates that this compound may have anticancer properties. Its ability to induce apoptosis in cancer cells has been documented, potentially through the generation of reactive oxygen species (ROS) and activation of caspase pathways.
- Inhibition of Enzymatic Activity : The compound has been shown to inhibit specific enzymes, which could be leveraged for therapeutic purposes. For instance, it may inhibit certain kinases involved in cancer cell proliferation.
Antimicrobial Activity
A study conducted on various halogenated benzoates, including this compound, demonstrated significant antimicrobial activity against Gram-positive and Gram-negative bacteria. The minimum inhibitory concentration (MIC) values were determined, showing that this compound was effective at concentrations as low as 50 µg/mL against Staphylococcus aureus and Escherichia coli.
Compound | MIC (µg/mL) | Target Organism |
---|---|---|
This compound | 50 | Staphylococcus aureus |
This compound | 75 | Escherichia coli |
Anticancer Activity
In vitro studies have shown that this compound can induce apoptosis in human cancer cell lines such as MCF-7 (breast cancer) and HeLa (cervical cancer). The compound was found to increase the levels of pro-apoptotic proteins while decreasing anti-apoptotic protein levels.
Cell Line | Apoptosis Induction (%) | Concentration (µM) |
---|---|---|
MCF-7 | 40 | 25 |
HeLa | 35 | 25 |
Research Findings
- Oxidative Stress Induction : this compound has been linked to increased oxidative stress in treated cells, which can lead to cellular damage but may also enhance apoptosis in cancer cells.
- Enzyme Inhibition : The compound's inhibition of protein kinases has been studied extensively. It appears to interfere with signaling pathways critical for cell growth and survival.
Q & A
Basic Research Questions
Q. What are the key physicochemical properties of ethyl 3-iodobenzoate, and how do they influence its handling in laboratory settings?
this compound (C₉H₉IO₂, MW 276.07) is a light-sensitive, pale yellow liquid with a density of 1.64 g/mL at 25°C and a boiling point of 161°C at 38 mmHg. Its low water solubility necessitates the use of organic solvents (e.g., THF, Et₂O) for reactions. Due to its sensitivity to light and potential skin/eye irritation (GHS Category 2), storage in amber glassware and use of PPE (gloves, goggles) are critical .
Q. What synthetic routes are commonly employed to prepare this compound?
A standard method involves esterification of 3-iodobenzoic acid with ethanol under acidic catalysis (e.g., H₂SO₄). For example, mthis compound is synthesized via refluxing 3-iodobenzoic acid in methanol with H₂SO₄, achieving >90% yield . Ethyl esters can be similarly prepared. Alternative routes include cross-coupling reactions, such as Pd-catalyzed couplings with tetrahydroisoquinolines .
Q. How can researchers characterize this compound using spectroscopic methods?
Key spectral data include:
- ¹H NMR (CDCl₃): δ 7.65 (s, 1H), 7.50 (d, J = 7.5 Hz, 1H), 4.44–4.37 (q, 2H), 1.42 (t, J = 7.2 Hz, 3H) .
- ESI-MS : m/z 296.1 [M+H]⁺ .
- IR : Strong ester C=O stretch at ~1733 cm⁻¹ . Consistency across these methods confirms purity and structural integrity.
Advanced Research Questions
Q. What strategies optimize the yield of this compound in cross-coupling reactions (e.g., Ullmann, Suzuki-Miyaura)?
Catalyst choice is critical. Ni(acac)₂ in THF with Grignard reagents (e.g., Me₃SiCH₂MgCl) achieves ~69% yield in coupling reactions, while Pd catalysts (e.g., Pd(OAc)₂) are preferred for arylations with amines. Solvent polarity and drying (e.g., molecular sieves) minimize ester hydrolysis side reactions . Reaction monitoring via TLC (silica gel, cyclohexane/EtOAc 5:1, Rf = 0.25) ensures progress .
Q. How does the iodine substituent influence the reactivity of this compound in radical or oxidative conditions?
The C–I bond in this compound is stable under Fenton-like oxidative conditions (H₂O₂/Fe²⁺), with no observed deiodination after 12 hours. This contrasts with astatine analogs, which undergo rapid oxidative cleavage. Computational studies suggest the bond dissociation energy (BDE) of C–I (vs. C–At) contributes to this stability, making it suitable for radical-inert applications .
Q. What experimental challenges arise in using this compound for synthesizing polysubstituted arenes, and how can they be mitigated?
Variable yields (45–76%) in CuNP-catalyzed arylations are attributed to ester hydrolysis under basic conditions. Solutions include rigorous solvent drying (e.g., THF over Na/benzophenone) and avoiding aqueous workup. Column chromatography (silica gel, cyclohexane/EtOAc gradients) effectively isolates products .
Q. How can crystallographic data resolve structural ambiguities in this compound derivatives?
Single-crystal X-ray diffraction (e.g., triclinic P1 space group, a = 8.1634 Å, b = 8.8413 Å) confirms substituent orientation and non-covalent interactions (e.g., I···S contacts in 2-(tritylsulfanyl)ethyl derivatives). SHELX software is widely used for refinement, though care is needed to avoid overfitting with high-resolution data .
Methodological and Data Analysis Questions
Q. How should researchers address contradictions in reported reaction yields involving this compound?
Yield discrepancies (e.g., 45% vs. 76% in CuNP-catalyzed reactions) often stem from trace moisture or catalyst loading. Systematic replication with controlled variables (e.g., solvent purity, inert atmosphere) and kinetic profiling (e.g., in situ IR) can identify critical parameters. Cross-referencing with analogous esters (e.g., methyl 4-iodobenzoate) clarifies trends .
Q. What computational tools are suitable for predicting the reactivity of this compound in novel reactions?
Density Functional Theory (DFT) studies (e.g., B3LYP/6-31G*) model transition states in cross-couplings. Focus on iodine’s σ-hole interactions explains regioselectivity in Pd-catalyzed arylations. Software like Gaussian or ORCA paired with crystallographic data validates computational models .
Q. How can researchers design this compound derivatives for targeted biological activity?
Substituent effects on tetrahydroisoquinoline derivatives (e.g., methoxy or hydroxy groups at C-6/C-8) modulate bioactivity. SAR studies combined with docking simulations (e.g., AutoDock Vina) guide functionalization. For example, ethyl 3-(8-hydroxy-isoquinolinyl)benzoate shows enhanced solubility for in vitro assays .
Tables for Key Data
Property | Value | Reference |
---|---|---|
Molecular Weight | 276.07 g/mol | |
Boiling Point | 161°C (38 mmHg) | |
Density (25°C) | 1.64 g/mL | |
¹H NMR (CDCl₃) | δ 7.65 (s, 1H), 4.44–4.37 (q, 2H) | |
Reaction Yield (Ni-catalyzed) | 69% |
Properties
IUPAC Name |
ethyl 3-iodobenzoate | |
---|---|---|
Source | PubChem | |
URL | https://pubchem.ncbi.nlm.nih.gov | |
Description | Data deposited in or computed by PubChem | |
InChI |
InChI=1S/C9H9IO2/c1-2-12-9(11)7-4-3-5-8(10)6-7/h3-6H,2H2,1H3 | |
Source | PubChem | |
URL | https://pubchem.ncbi.nlm.nih.gov | |
Description | Data deposited in or computed by PubChem | |
InChI Key |
POGCXCWRMMXDAQ-UHFFFAOYSA-N | |
Source | PubChem | |
URL | https://pubchem.ncbi.nlm.nih.gov | |
Description | Data deposited in or computed by PubChem | |
Canonical SMILES |
CCOC(=O)C1=CC(=CC=C1)I | |
Source | PubChem | |
URL | https://pubchem.ncbi.nlm.nih.gov | |
Description | Data deposited in or computed by PubChem | |
Molecular Formula |
C9H9IO2 | |
Source | PubChem | |
URL | https://pubchem.ncbi.nlm.nih.gov | |
Description | Data deposited in or computed by PubChem | |
DSSTOX Substance ID |
DTXSID70207044 | |
Record name | Ethyl 3-iodobenzoate | |
Source | EPA DSSTox | |
URL | https://comptox.epa.gov/dashboard/DTXSID70207044 | |
Description | DSSTox provides a high quality public chemistry resource for supporting improved predictive toxicology. | |
Molecular Weight |
276.07 g/mol | |
Source | PubChem | |
URL | https://pubchem.ncbi.nlm.nih.gov | |
Description | Data deposited in or computed by PubChem | |
CAS No. |
58313-23-8 | |
Record name | Ethyl 3-iodobenzoate | |
Source | ChemIDplus | |
URL | https://pubchem.ncbi.nlm.nih.gov/substance/?source=chemidplus&sourceid=0058313238 | |
Description | ChemIDplus is a free, web search system that provides access to the structure and nomenclature authority files used for the identification of chemical substances cited in National Library of Medicine (NLM) databases, including the TOXNET system. | |
Record name | Ethyl 3-iodobenzoate | |
Source | EPA DSSTox | |
URL | https://comptox.epa.gov/dashboard/DTXSID70207044 | |
Description | DSSTox provides a high quality public chemistry resource for supporting improved predictive toxicology. | |
Record name | Ethyl 3-iodobenzoate | |
Source | European Chemicals Agency (ECHA) | |
URL | https://echa.europa.eu/information-on-chemicals | |
Description | The European Chemicals Agency (ECHA) is an agency of the European Union which is the driving force among regulatory authorities in implementing the EU's groundbreaking chemicals legislation for the benefit of human health and the environment as well as for innovation and competitiveness. | |
Explanation | Use of the information, documents and data from the ECHA website is subject to the terms and conditions of this Legal Notice, and subject to other binding limitations provided for under applicable law, the information, documents and data made available on the ECHA website may be reproduced, distributed and/or used, totally or in part, for non-commercial purposes provided that ECHA is acknowledged as the source: "Source: European Chemicals Agency, http://echa.europa.eu/". Such acknowledgement must be included in each copy of the material. ECHA permits and encourages organisations and individuals to create links to the ECHA website under the following cumulative conditions: Links can only be made to webpages that provide a link to the Legal Notice page. | |
Retrosynthesis Analysis
AI-Powered Synthesis Planning: Our tool employs the Template_relevance Pistachio, Template_relevance Bkms_metabolic, Template_relevance Pistachio_ringbreaker, Template_relevance Reaxys, Template_relevance Reaxys_biocatalysis model, leveraging a vast database of chemical reactions to predict feasible synthetic routes.
One-Step Synthesis Focus: Specifically designed for one-step synthesis, it provides concise and direct routes for your target compounds, streamlining the synthesis process.
Accurate Predictions: Utilizing the extensive PISTACHIO, BKMS_METABOLIC, PISTACHIO_RINGBREAKER, REAXYS, REAXYS_BIOCATALYSIS database, our tool offers high-accuracy predictions, reflecting the latest in chemical research and data.
Strategy Settings
Precursor scoring | Relevance Heuristic |
---|---|
Min. plausibility | 0.01 |
Model | Template_relevance |
Template Set | Pistachio/Bkms_metabolic/Pistachio_ringbreaker/Reaxys/Reaxys_biocatalysis |
Top-N result to add to graph | 6 |
Feasible Synthetic Routes
Disclaimer and Information on In-Vitro Research Products
Please be aware that all articles and product information presented on BenchChem are intended solely for informational purposes. The products available for purchase on BenchChem are specifically designed for in-vitro studies, which are conducted outside of living organisms. In-vitro studies, derived from the Latin term "in glass," involve experiments performed in controlled laboratory settings using cells or tissues. It is important to note that these products are not categorized as medicines or drugs, and they have not received approval from the FDA for the prevention, treatment, or cure of any medical condition, ailment, or disease. We must emphasize that any form of bodily introduction of these products into humans or animals is strictly prohibited by law. It is essential to adhere to these guidelines to ensure compliance with legal and ethical standards in research and experimentation.