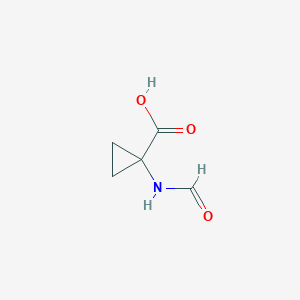
1-Formamidocyclopropane-1-carboxylic acid
- Click on QUICK INQUIRY to receive a quote from our team of experts.
- With the quality product at a COMPETITIVE price, you can focus more on your research.
Overview
Description
1-Formamidocyclopropane-1-carboxylic acid is a chemical compound with the molecular formula C₅H₇NO₃ It is a derivative of cyclopropane, characterized by the presence of a formamido group and a carboxylic acid group attached to the cyclopropane ring
Preparation Methods
The synthesis of 1-formamidocyclopropane-1-carboxylic acid can be achieved through several methods:
Alkylation of Glycine Equivalents: This method involves the alkylation of glycine equivalents with 1,2-electrophiles.
Intramolecular Cyclization: This approach uses γ-substituted amino acid derivatives to form the cyclopropane ring.
Cyclopropanation of Alkenes: This method involves the cyclopropanation of alkenes using diazo compounds, ylides, or carbene intermediates.
Chemical Reactions Analysis
1-Formamidocyclopropane-1-carboxylic acid undergoes various chemical reactions:
Oxidation: The compound can be oxidized under specific conditions, although detailed reaction pathways are not extensively documented.
Reduction: Reduction reactions can convert the formamido group to an amino group.
Substitution: The carboxylic acid group can participate in nucleophilic substitution reactions, forming esters or amides.
Common reagents used in these reactions include thionyl chloride for forming acid chlorides and various reducing agents for reduction reactions . The major products formed depend on the specific reaction conditions and reagents used.
Scientific Research Applications
1-Formamidocyclopropane-1-carboxylic acid has several applications in scientific research:
Chemistry: It serves as a building block for synthesizing more complex molecules and studying reaction mechanisms.
Medicine: Research is ongoing to explore its potential therapeutic applications, although specific uses are not yet well-established.
Mechanism of Action
The mechanism of action of 1-formamidocyclopropane-1-carboxylic acid involves its interaction with various molecular targets and pathways:
Comparison with Similar Compounds
1-Formamidocyclopropane-1-carboxylic acid can be compared with other similar compounds:
1-Aminocyclopropane-1-carboxylic Acid: This compound is a direct precursor of ethylene and plays a significant role in plant physiology.
Cyclopropane Derivatives: Other cyclopropane derivatives, such as 1-aminocyclopropane-1-carboxylic acid, share structural similarities but differ in functional groups and biological activities.
Biological Activity
1-Formamidocyclopropane-1-carboxylic acid (FCCA) is a cyclopropane derivative that has garnered attention due to its potential biological activities. This compound, related to 1-aminocyclopropane-1-carboxylic acid (ACC), plays a significant role in various biochemical pathways, particularly in plants where it is involved in ethylene biosynthesis. This article explores the biological activity of FCCA, highlighting its mechanisms, effects, and potential applications based on diverse research findings.
FCCA is structurally similar to ACC and is hypothesized to influence ethylene production in plants. Ethylene is a critical plant hormone that regulates growth, development, and responses to environmental stimuli. The conversion of ACC to ethylene is catalyzed by the enzyme ACC oxidase, which requires FCCA as a substrate under certain conditions. The detailed mechanism involves the binding of FCCA to the active site of ACC oxidase, facilitating the conversion process.
Key Enzymatic Pathways
- ACC Synthase (ACS) : FCCA may modulate the activity of ACS, which catalyzes the formation of ACC from S-adenosylmethionine (SAM).
- ACC Oxidase (ACCO) : FCCA can act as an inhibitor or enhancer of ACCO activity, influencing ethylene production rates depending on its concentration and environmental factors.
Biological Effects
The biological effects of FCCA can be categorized into several key areas:
1. Plant Growth Regulation
FCCA has been shown to affect various growth parameters in plants:
- Root Development : Enhanced root elongation and branching.
- Fruit Ripening : Accelerated ripening processes through increased ethylene production.
- Stress Responses : Modulation of plant responses to biotic and abiotic stresses.
2. Antimicrobial Activity
Recent studies indicate that FCCA exhibits antimicrobial properties against certain pathogens:
- Bacterial Inhibition : Inhibits growth of specific bacteria by disrupting their metabolic pathways.
- Fungal Resistance : Enhances resistance in plants against fungal infections by promoting ethylene-mediated defense mechanisms.
Research Findings and Case Studies
Study | Findings | Methodology |
---|---|---|
Honma & Shimomura (1978) | Identified metabolic pathways involving FCCA in plants | Biochemical assays on plant tissues |
Boller et al. (1979) | Demonstrated the role of FCCA in ethylene biosynthesis | Enzyme kinetics studies |
Tsuchisaka et al. (2009) | Explored tissue-specific expression patterns of ACS influenced by FCCA | Genetic analysis using knockout mutants |
Structure-Activity Relationship (SAR)
The structure-activity relationship studies have highlighted how modifications to the FCCA structure can influence its biological activity:
- Functional Groups : The presence of specific functional groups enhances binding affinity to target enzymes.
- Steric Effects : Alterations in molecular geometry affect the inhibitory or stimulatory effects on ACC oxidase.
Properties
CAS No. |
42303-43-5 |
---|---|
Molecular Formula |
C5H7NO3 |
Molecular Weight |
129.11 g/mol |
IUPAC Name |
1-formamidocyclopropane-1-carboxylic acid |
InChI |
InChI=1S/C5H7NO3/c7-3-6-5(1-2-5)4(8)9/h3H,1-2H2,(H,6,7)(H,8,9) |
InChI Key |
UPIUTCMVUPFJTQ-UHFFFAOYSA-N |
Canonical SMILES |
C1CC1(C(=O)O)NC=O |
Origin of Product |
United States |
Disclaimer and Information on In-Vitro Research Products
Please be aware that all articles and product information presented on BenchChem are intended solely for informational purposes. The products available for purchase on BenchChem are specifically designed for in-vitro studies, which are conducted outside of living organisms. In-vitro studies, derived from the Latin term "in glass," involve experiments performed in controlled laboratory settings using cells or tissues. It is important to note that these products are not categorized as medicines or drugs, and they have not received approval from the FDA for the prevention, treatment, or cure of any medical condition, ailment, or disease. We must emphasize that any form of bodily introduction of these products into humans or animals is strictly prohibited by law. It is essential to adhere to these guidelines to ensure compliance with legal and ethical standards in research and experimentation.