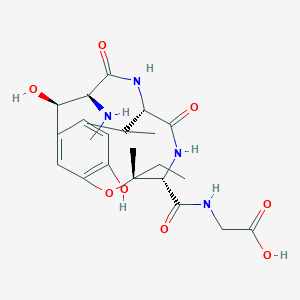
Ustiloxin D
- Click on QUICK INQUIRY to receive a quote from our team of experts.
- With the quality product at a COMPETITIVE price, you can focus more on your research.
Overview
Description
Ustiloxin D is a toxic cyclic peptide produced by the pathogenic fungus Ustilaginoidea virens, which causes rice false smut disease.
Preparation Methods
Synthetic Routes and Reaction Conditions
The total synthesis of ustiloxin D has been achieved through various methods. One notable approach involves a convergent strategy incorporating an asymmetric allylic alkylation to construct the tert-alkyl aryl ether linkage between the dopa and isoleucine residues. This method also utilizes an ammonia Ugi reaction to rapidly convert a β-hydroxydopa derivative to a linear tripeptide, followed by cyclization and global deprotection .
Another method involves a 31-step synthesis utilizing a nucleophilic aromatic substitution (SNAr) reaction to construct the chiral alkyl-aryl ether . This approach has been refined over time to reduce the number of steps and improve efficiency.
Industrial Production Methods
Industrial production of this compound is not well-documented, likely due to its complex structure and the challenges associated with its synthesis
Chemical Reactions Analysis
Types of Reactions
Ustiloxin D undergoes various chemical reactions, including:
Oxidation: This reaction can modify the functional groups within the molecule, potentially altering its biological activity.
Reduction: Reduction reactions can be used to modify specific functional groups, such as reducing ketones to alcohols.
Substitution: Nucleophilic substitution reactions, such as the SNAr reaction, are crucial in the synthesis of this compound.
Common Reagents and Conditions
Oxidation: Common oxidizing agents include potassium permanganate and chromium trioxide.
Reduction: Reducing agents such as lithium aluminum hydride and sodium borohydride are often used.
Substitution: Reagents like sodium hydride and various alkyl halides are used in nucleophilic substitution reactions.
Major Products
The major products formed from these reactions depend on the specific conditions and reagents used. For example, oxidation can produce ketones or carboxylic acids, while reduction can yield alcohols.
Scientific Research Applications
Ustiloxin D has several scientific research applications:
Chemistry: It serves as a model compound for studying complex peptide synthesis and the development of new synthetic methodologies.
Biology: this compound is used to study the mechanisms of microtubule inhibition and cell division.
Medicine: Due to its antimitotic properties, this compound is investigated as a potential antitumor agent.
Mechanism of Action
Ustiloxin D exerts its effects by binding to the vinca/rhizoxin site on tubulin, inhibiting the assembly of α, β-tubulin dimers into microtubules. This inhibition disrupts cell division, leading to antimitotic activity . The compound’s structure, particularly the chiral tertiary alkyl-aryl ether linkage, is crucial for its biological activity.
Comparison with Similar Compounds
Similar Compounds
Ustiloxin A: Another member of the ustiloxin family, known for its strong inhibition of seed germination and similar antimitotic activity.
Ustiloxin B: Similar to ustiloxin A, but with slight variations in its peptide sequence and biological activity.
Ustiloxin F: Shares the cyclic core structure with ustiloxin D but differs in its side chain composition.
Uniqueness
This compound is unique due to its specific chiral tertiary alkyl-aryl ether linkage, which is essential for its potent biological activity. This structural feature distinguishes it from other ustiloxins and contributes to its effectiveness as an antimitotic agent .
Properties
CAS No. |
158243-18-6 |
---|---|
Molecular Formula |
C23H34N4O8 |
Molecular Weight |
494.5 g/mol |
IUPAC Name |
2-[[(3R,4S,7S,10S,11R)-3-ethyl-11,15-dihydroxy-3-methyl-10-(methylamino)-6,9-dioxo-7-propan-2-yl-2-oxa-5,8-diazabicyclo[10.3.1]hexadeca-1(15),12(16),13-triene-4-carbonyl]amino]acetic acid |
InChI |
InChI=1S/C23H34N4O8/c1-6-23(4)19(22(34)25-10-15(29)30)27-20(32)16(11(2)3)26-21(33)17(24-5)18(31)12-7-8-13(28)14(9-12)35-23/h7-9,11,16-19,24,28,31H,6,10H2,1-5H3,(H,25,34)(H,26,33)(H,27,32)(H,29,30)/t16-,17-,18+,19+,23+/m0/s1 |
InChI Key |
GDXLZSYACWZHOC-ZNRYNLAGSA-N |
SMILES |
CCC1(C(NC(=O)C(NC(=O)C(C(C2=CC(=C(C=C2)O)O1)O)NC)C(C)C)C(=O)NCC(=O)O)C |
Isomeric SMILES |
CC[C@@]1([C@H](NC(=O)[C@@H](NC(=O)[C@H]([C@@H](C2=CC(=C(C=C2)O)O1)O)NC)C(C)C)C(=O)NCC(=O)O)C |
Canonical SMILES |
CCC1(C(NC(=O)C(NC(=O)C(C(C2=CC(=C(C=C2)O)O1)O)NC)C(C)C)C(=O)NCC(=O)O)C |
Key on ui other cas no. |
158243-18-6 |
Synonyms |
Ustiloxin D |
Origin of Product |
United States |
Retrosynthesis Analysis
AI-Powered Synthesis Planning: Our tool employs the Template_relevance Pistachio, Template_relevance Bkms_metabolic, Template_relevance Pistachio_ringbreaker, Template_relevance Reaxys, Template_relevance Reaxys_biocatalysis model, leveraging a vast database of chemical reactions to predict feasible synthetic routes.
One-Step Synthesis Focus: Specifically designed for one-step synthesis, it provides concise and direct routes for your target compounds, streamlining the synthesis process.
Accurate Predictions: Utilizing the extensive PISTACHIO, BKMS_METABOLIC, PISTACHIO_RINGBREAKER, REAXYS, REAXYS_BIOCATALYSIS database, our tool offers high-accuracy predictions, reflecting the latest in chemical research and data.
Strategy Settings
Precursor scoring | Relevance Heuristic |
---|---|
Min. plausibility | 0.01 |
Model | Template_relevance |
Template Set | Pistachio/Bkms_metabolic/Pistachio_ringbreaker/Reaxys/Reaxys_biocatalysis |
Top-N result to add to graph | 6 |
Feasible Synthetic Routes
Disclaimer and Information on In-Vitro Research Products
Please be aware that all articles and product information presented on BenchChem are intended solely for informational purposes. The products available for purchase on BenchChem are specifically designed for in-vitro studies, which are conducted outside of living organisms. In-vitro studies, derived from the Latin term "in glass," involve experiments performed in controlled laboratory settings using cells or tissues. It is important to note that these products are not categorized as medicines or drugs, and they have not received approval from the FDA for the prevention, treatment, or cure of any medical condition, ailment, or disease. We must emphasize that any form of bodily introduction of these products into humans or animals is strictly prohibited by law. It is essential to adhere to these guidelines to ensure compliance with legal and ethical standards in research and experimentation.