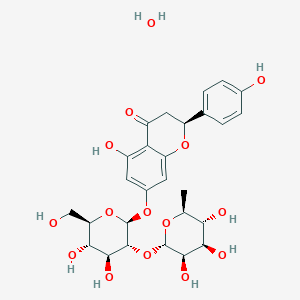
Naringin hydrate
Overview
Description
Naringin hydrate is a flavanone-7-O-glycoside between the flavanone naringenin and the disaccharide neohesperidose. It is a naturally occurring compound found predominantly in citrus fruits, especially grapefruits, where it contributes to the fruit’s bitter taste . This compound is known for its various biological activities, including antioxidant, anti-inflammatory, and anticancer properties .
Mechanism of Action
Naringin hydrate is a bioactive compound found in citrus fruits, known for its wide range of pharmacological properties . This article aims to provide an in-depth understanding of its mechanism of action.
Target of Action
this compound primarily targets multiple cellular signaling pathways . It interacts with various molecules such as the HTH-type transcriptional regulator TtgR, Estrogen receptor alpha, Aldo-keto reductase family 1 member C1, Cytochrome P450 1B1, KAT8 regulatory NSL complex subunit 3, Sex hormone-binding globulin, and Cytochrome P450 19A1 .
Mode of Action
this compound exhibits pleiotropic anticancer activities by modulating different cellular signaling pathways, suppressing cytokine and growth factor production, and arresting the cell cycle . It also decreases the expression of Snail1/SMAD2, a key factor in cellular proliferation .
Biochemical Pathways
this compound affects various biochemical pathways. It has been reported to regulate lipid metabolism, contributing to diabetes control . It also promotes cell proliferation and bone differentiation by increasing the OPG:RANKL ratio, enhancing BMP and osteocalcin expression, and decreasing HMG-CoA, NF-jB, and ERK expression .
Pharmacokinetics
The pharmacokinetics of this compound involves its absorption, distribution, metabolism, and excretion (ADME). It has been observed that this compound shows an insignificant influence of high-fat diet and insignificant accumulation of the drugs after multiple dosing . It’s important to note that this compound has a weakly basic nature and is slightly soluble in water, which can affect its bioavailability .
Result of Action
The molecular and cellular effects of this compound’s action are diverse. It has been reported to have antioxidant and antiandrogenic properties, as well as the ability to protect from inflammation and cancer . It can suppress cancer development in various body parts, alleviating the conditions of cancer patients . Moreover, it has been found to decrease insulin resistance, lower blood sugar levels, and improve cell absorption of glucose .
Action Environment
The action, efficacy, and stability of this compound can be influenced by various environmental factors. For instance, factors such as ultraviolet rays, pollution, radiation, smoking, and stress can lead to internal changes, such as oxidative stress, impaired apoptosis, and increased rates of genetic mutations . These factors can potentially affect the effectiveness of this compound.
Biochemical Analysis
Biochemical Properties
Naringin hydrate plays a significant role in various biochemical reactions. It interacts with several enzymes, proteins, and other biomolecules. One of the key enzymes it interacts with is naringinase, which hydrolyzes naringin into rhamnose and prunin. Prunin is further hydrolyzed by D-glucosidase into glucose and naringenin . This compound also exhibits antioxidant properties by scavenging free radicals and reducing oxidative stress. Additionally, it has been shown to inhibit enzymes such as cyclooxygenase-2 (COX-2) and inducible nitric oxide synthase (iNOS), which are involved in inflammatory processes .
Cellular Effects
This compound exerts various effects on different types of cells and cellular processes. It has been shown to influence cell function by modulating cell signaling pathways, gene expression, and cellular metabolism. For instance, this compound can activate the AMP-activated protein kinase (AMPK) pathway, leading to enhanced glucose uptake and improved insulin sensitivity . It also affects the expression of genes involved in apoptosis, such as Bcl-2 and Bax, thereby promoting cell survival and reducing cell death . Furthermore, this compound has been reported to inhibit the proliferation of cancer cells by arresting the cell cycle and inducing apoptosis .
Molecular Mechanism
The molecular mechanism of this compound involves several pathways and interactions at the molecular level. This compound binds to various biomolecules, including proteins and enzymes, to exert its effects. It has been shown to inhibit the activity of nuclear factor-kappa B (NF-κB), a transcription factor involved in inflammation and cancer progression . Additionally, this compound can activate the p38 mitogen-activated protein kinase (MAPK) pathway, leading to the induction of apoptosis in cancer cells . It also modulates the expression of genes involved in oxidative stress, such as superoxide dismutase (SOD) and catalase, thereby enhancing the antioxidant defense system .
Temporal Effects in Laboratory Settings
In laboratory settings, the effects of this compound have been studied over time to understand its stability, degradation, and long-term effects on cellular function. This compound has been found to be relatively stable under various conditions, with minimal degradation observed over time . Long-term studies have shown that this compound can maintain its antioxidant and anti-inflammatory properties even after prolonged exposure . Additionally, in vitro and in vivo studies have demonstrated that this compound can exert sustained effects on cellular function, including the inhibition of cancer cell proliferation and the reduction of oxidative stress .
Dosage Effects in Animal Models
The effects of this compound vary with different dosages in animal models. Studies have shown that low to moderate doses of this compound can exert beneficial effects, such as reducing inflammation and oxidative stress . At high doses, this compound may exhibit toxic or adverse effects, including hepatotoxicity and nephrotoxicity . Threshold effects have also been observed, where the beneficial effects of this compound plateau at higher doses, indicating that optimal dosing is crucial for maximizing its therapeutic potential .
Metabolic Pathways
This compound is involved in several metabolic pathways, including its conversion to naringenin by intestinal microflora . Naringenin, the active metabolite of this compound, undergoes further metabolism, including hydroxylation, hydrogenation, dehydrogenation, and acetylation . These metabolic processes are catalyzed by various enzymes, such as cytochrome P450 enzymes, in the liver and kidneys . This compound also influences metabolic flux and metabolite levels, contributing to its overall bioactivity .
Transport and Distribution
The transport and distribution of this compound within cells and tissues involve several mechanisms. This compound is absorbed in the intestines and transported to various tissues via the bloodstream . It interacts with transporters and binding proteins, such as albumin, to facilitate its distribution . This compound has been shown to accumulate in specific tissues, including the liver, kidneys, and adipose tissue, where it exerts its biological effects . Its localization and accumulation are influenced by factors such as tissue permeability and binding affinity .
Subcellular Localization
This compound exhibits specific subcellular localization, which affects its activity and function. It has been found to localize in various cellular compartments, including the cytoplasm, mitochondria, and nucleus . The subcellular localization of this compound is influenced by targeting signals and post-translational modifications, which direct it to specific compartments or organelles . For instance, this compound can accumulate in the mitochondria, where it enhances mitochondrial function and reduces oxidative stress . Its localization in the nucleus allows it to modulate gene expression and regulate cellular processes .
Preparation Methods
Synthetic Routes and Reaction Conditions
Naringin hydrate can be synthesized through several methods. One conventional method involves the extraction of naringin from citrus fruits using an alkaline solution, such as lime or sodium hydroxide, followed by precipitation in cold acidic water . Another method involves the use of nanotechnological strategies to improve the bioavailability and stability of naringin .
Industrial Production Methods
In industrial settings, this compound is often extracted from grapefruit peels and other citrus fruit residues. The extraction process typically involves the use of solvents like ethanol or methanol, followed by purification steps to isolate the compound .
Chemical Reactions Analysis
Types of Reactions
Naringin hydrate undergoes various chemical reactions, including:
Oxidation: Naringin can be oxidized to form naringenin, its aglycone form.
Hydrolysis: Enzymatic hydrolysis of naringin by naringinase results in the formation of naringenin and sugars.
Reduction: Naringin can be reduced to form dihydro-naringin under specific conditions.
Common Reagents and Conditions
Oxidation: Common oxidizing agents include hydrogen peroxide and potassium permanganate.
Hydrolysis: Naringinase enzyme is commonly used for hydrolysis reactions.
Reduction: Reducing agents like sodium borohydride can be used.
Major Products Formed
Oxidation: Naringenin
Hydrolysis: Naringenin and sugars (rhamnose and glucose)
Reduction: Dihydro-naringin
Scientific Research Applications
Naringin hydrate has a wide range of scientific research applications:
Comparison with Similar Compounds
Similar Compounds
Naringenin: The aglycone form of naringin, lacking the sugar moieties.
Hesperidin: Another flavonoid glycoside found in citrus fruits, with similar antioxidant and anti-inflammatory properties.
Rutin: A flavonoid glycoside found in various plants, known for its antioxidant and anti-inflammatory effects.
Uniqueness of Naringin Hydrate
This compound is unique due to its specific glycoside structure, which includes both rhamnose and glucose units. This structure contributes to its distinct biological activities and its role in the bitterness of grapefruit .
Properties
IUPAC Name |
(2S)-7-[(2S,3R,4S,5S,6R)-4,5-dihydroxy-6-(hydroxymethyl)-3-[(2S,3R,4R,5R,6S)-3,4,5-trihydroxy-6-methyloxan-2-yl]oxyoxan-2-yl]oxy-5-hydroxy-2-(4-hydroxyphenyl)-2,3-dihydrochromen-4-one;hydrate | |
---|---|---|
Source | PubChem | |
URL | https://pubchem.ncbi.nlm.nih.gov | |
Description | Data deposited in or computed by PubChem | |
InChI |
InChI=1S/C27H32O14.H2O/c1-10-20(32)22(34)24(36)26(37-10)41-25-23(35)21(33)18(9-28)40-27(25)38-13-6-14(30)19-15(31)8-16(39-17(19)7-13)11-2-4-12(29)5-3-11;/h2-7,10,16,18,20-30,32-36H,8-9H2,1H3;1H2/t10-,16-,18+,20-,21+,22+,23-,24+,25+,26-,27+;/m0./s1 | |
Source | PubChem | |
URL | https://pubchem.ncbi.nlm.nih.gov | |
Description | Data deposited in or computed by PubChem | |
InChI Key |
CPNHAWBPPPWRLN-OSSFAINNSA-N | |
Source | PubChem | |
URL | https://pubchem.ncbi.nlm.nih.gov | |
Description | Data deposited in or computed by PubChem | |
Canonical SMILES |
CC1C(C(C(C(O1)OC2C(C(C(OC2OC3=CC(=C4C(=O)CC(OC4=C3)C5=CC=C(C=C5)O)O)CO)O)O)O)O)O.O | |
Source | PubChem | |
URL | https://pubchem.ncbi.nlm.nih.gov | |
Description | Data deposited in or computed by PubChem | |
Isomeric SMILES |
C[C@H]1[C@@H]([C@H]([C@H]([C@@H](O1)O[C@@H]2[C@H]([C@@H]([C@H](O[C@H]2OC3=CC(=C4C(=O)C[C@H](OC4=C3)C5=CC=C(C=C5)O)O)CO)O)O)O)O)O.O | |
Source | PubChem | |
URL | https://pubchem.ncbi.nlm.nih.gov | |
Description | Data deposited in or computed by PubChem | |
Molecular Formula |
C27H34O15 | |
Source | PubChem | |
URL | https://pubchem.ncbi.nlm.nih.gov | |
Description | Data deposited in or computed by PubChem | |
Molecular Weight |
598.5 g/mol | |
Source | PubChem | |
URL | https://pubchem.ncbi.nlm.nih.gov | |
Description | Data deposited in or computed by PubChem | |
Retrosynthesis Analysis
AI-Powered Synthesis Planning: Our tool employs the Template_relevance Pistachio, Template_relevance Bkms_metabolic, Template_relevance Pistachio_ringbreaker, Template_relevance Reaxys, Template_relevance Reaxys_biocatalysis model, leveraging a vast database of chemical reactions to predict feasible synthetic routes.
One-Step Synthesis Focus: Specifically designed for one-step synthesis, it provides concise and direct routes for your target compounds, streamlining the synthesis process.
Accurate Predictions: Utilizing the extensive PISTACHIO, BKMS_METABOLIC, PISTACHIO_RINGBREAKER, REAXYS, REAXYS_BIOCATALYSIS database, our tool offers high-accuracy predictions, reflecting the latest in chemical research and data.
Strategy Settings
Precursor scoring | Relevance Heuristic |
---|---|
Min. plausibility | 0.01 |
Model | Template_relevance |
Template Set | Pistachio/Bkms_metabolic/Pistachio_ringbreaker/Reaxys/Reaxys_biocatalysis |
Top-N result to add to graph | 6 |
Feasible Synthetic Routes
Disclaimer and Information on In-Vitro Research Products
Please be aware that all articles and product information presented on BenchChem are intended solely for informational purposes. The products available for purchase on BenchChem are specifically designed for in-vitro studies, which are conducted outside of living organisms. In-vitro studies, derived from the Latin term "in glass," involve experiments performed in controlled laboratory settings using cells or tissues. It is important to note that these products are not categorized as medicines or drugs, and they have not received approval from the FDA for the prevention, treatment, or cure of any medical condition, ailment, or disease. We must emphasize that any form of bodily introduction of these products into humans or animals is strictly prohibited by law. It is essential to adhere to these guidelines to ensure compliance with legal and ethical standards in research and experimentation.