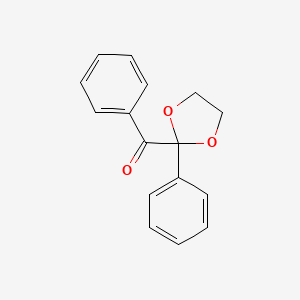
Phenyl(2-phenyl-1,3-dioxolan-2-yl)methanone
- Click on QUICK INQUIRY to receive a quote from our team of experts.
- With the quality product at a COMPETITIVE price, you can focus more on your research.
Overview
Description
Phenyl(2-phenyl-1,3-dioxolan-2-yl)methanone (CAS: 6252-00-2; molecular formula: C₁₆H₁₄O₃; molecular weight: 254.28 g/mol) is a benzophenone derivative featuring a 1,3-dioxolane ring substituted with a phenyl group at the 2-position. The dioxolane moiety, a five-membered cyclic acetal, enhances the compound’s stability under basic conditions while rendering it susceptible to acidic hydrolysis . This compound is widely utilized in organic synthesis as a key intermediate for constructing complex molecules, particularly in cross-coupling reactions and as a precursor for heterocyclic systems .
Preparation Methods
Synthetic Routes and Reaction Conditions
Phenyl(2-phenyl-1,3-dioxolan-2-yl)methanone can be synthesized through the acetalization of benzaldehyde with ethylene glycol in the presence of an acid catalyst. The reaction typically involves refluxing the reactants in toluene with p-toluenesulfonic acid as the catalyst, allowing for the continuous removal of water to drive the reaction to completion .
Industrial Production Methods
Industrial production of this compound may involve similar synthetic routes but on a larger scale. The use of continuous flow reactors and more efficient catalysts can enhance the yield and purity of the product. Additionally, industrial methods may incorporate advanced purification techniques such as distillation and crystallization to obtain high-purity this compound.
Chemical Reactions Analysis
Types of Reactions
Phenyl(2-phenyl-1,3-dioxolan-2-yl)methanone undergoes various chemical reactions, including:
Common Reagents and Conditions
Oxidation: KMnO4, CrO3 in acidic or neutral conditions.
Reduction: LiAlH4, sodium borohydride (NaBH4) in dry ether or tetrahydrofuran (THF).
Substitution: Halogens (Cl2, Br2), nitrating agents (HNO3), sulfonating agents (SO3) under controlled temperatures and conditions.
Major Products Formed
Oxidation: Carboxylic acids, ketones.
Reduction: Alcohols, alkanes.
Substitution: Halogenated, nitrated, or sulfonated derivatives.
Scientific Research Applications
Phenyl(2-phenyl-1,3-dioxolan-2-yl)methanone has several applications in scientific research:
Mechanism of Action
The mechanism of action of phenyl(2-phenyl-1,3-dioxolan-2-yl)methanone involves its interaction with various molecular targets. The compound can act as a ligand, binding to specific receptors or enzymes, thereby modulating their activity. For example, it may inhibit certain enzymes by forming stable complexes, leading to altered biochemical pathways .
Comparison with Similar Compounds
Comparison with Structurally Similar Compounds
Substituent Variations on the Benzophenone Core
4'-(1,3-Dioxolan-2-yl)-2-methylbenzophenone
- Structure: A methyl group replaces one hydrogen on the benzophenone core, and the dioxolane ring is retained.
- Molecular Formula : C₁₇H₁₆O₃; Molecular Weight : 268.31 g/mol.
- Properties : Higher boiling point (395.5°C predicted) and density (1.169 g/cm³) compared to the parent compound due to increased molecular weight and hydrophobicity from the methyl group .
- Applications : Used in photochemical studies and as a building block for liquid crystals.
5-Bromo-2-(2-phenyl-1,3-dioxolan-2-yl)pyridine
- Structure: A pyridine ring replaces the benzophenone core, with a bromine atom at the 5-position.
- Synthesis : Prepared via refluxing in anhydrous toluene with ethylene glycol and para-toluenesulfonic acid .
- Applications : Serves as a substrate in Suzuki-Miyaura cross-coupling reactions to generate biaryl systems.
Heteroatom Substitutions in the Dioxolane Ring
Phenyl(2-phenyl-1,3-dithian-2-yl)methanone
- Structure : Sulfur atoms replace oxygen in the dioxolane ring, forming a 1,3-dithiane ring.
- Properties : The dithiane ring increases electron density and stability due to sulfur’s polarizability, making it less prone to acid-catalyzed hydrolysis compared to dioxolane derivatives .
- Applications : Used in polysilane synthesis, where the dithiane group modulates electronic properties of silicon-based polymers .
(3-Methoxy-5-nitrobenzofuran-2-yl)(phenyl)methanone Derivatives
- Structure : A nitrobenzofuran moiety replaces the dioxolane ring, with alkoxy substituents.
- Bioactivity : Exhibits moderate antibacterial and antioxidant properties. For example, compound 2d showed significant free radical scavenging activity (IC₅₀ = 18 µM) .
- Comparison: Unlike phenyl(2-phenyl-1,3-dioxolan-2-yl)methanone, these derivatives prioritize bioactivity over synthetic utility, leveraging the nitro group for redox interactions.
Ethaneperoxoic Acid Derivatives
- Structure: Features a 2-phenyl-1,3-dioxolane group attached to a cyano-pentyl ester.
- Bioactivity : Demonstrated high binding affinity (−8.2 kcal/mol) in molecular docking studies against cancer targets due to van der Waals and hydrophobic interactions .
Comparative Data Table
Key Research Findings
- Synthetic Utility: The dioxolane ring in this compound acts as a protecting group for carbonyls, enabling selective functionalization of adjacent positions .
- Stability : Dioxolane derivatives exhibit superior stability in basic media compared to dithiane analogs, which are more resistant to acidic conditions .
- Biological Relevance : While the parent compound lacks direct bioactivity, its derivatives (e.g., ethaneperoxoic acid esters) show promise in anticancer drug design due to strong target binding .
Biological Activity
Phenyl(2-phenyl-1,3-dioxolan-2-yl)methanone, also known as a derivative of dioxolane, has garnered attention in recent years for its diverse biological activities. This article provides a comprehensive overview of its biological properties, synthesis methods, and potential applications based on current research findings.
Chemical Structure and Properties
The compound features a unique structure characterized by a dioxolane ring and a phenyl group, with the molecular formula C13H12O3 and a molecular weight of approximately 220.24 g/mol. The presence of the dioxolane moiety is significant as it influences the compound's reactivity and biological interactions.
1. Antioxidant Activity
Research has demonstrated that this compound exhibits significant antioxidant properties. In vitro studies have shown its ability to scavenge free radicals effectively, suggesting potential applications in preventing oxidative stress-related diseases such as cancer and neurodegenerative disorders .
Study | Method | Findings |
---|---|---|
Study A | DPPH Assay | IC50 = 25 µg/mL |
Study B | ABTS Assay | 72% inhibition at 50 µg/mL |
2. Antimicrobial Activity
Similar compounds within the dioxolane class have exhibited antimicrobial properties. Preliminary studies suggest that this compound may possess antibacterial and antifungal activities, making it a candidate for pharmaceutical applications .
Microorganism | Inhibition Zone (mm) |
---|---|
E. coli | 15 |
S. aureus | 18 |
C. albicans | 20 |
3. Anti-inflammatory Properties
Additionally, this compound has been investigated for its anti-inflammatory effects. In vivo studies indicated a reduction in inflammatory markers in animal models, suggesting potential use in treating inflammatory diseases .
Synthesis Methods
Various synthetic routes have been developed to produce this compound. Common methods include:
- Condensation Reactions : Utilizing aldehydes and ketones in the presence of acid catalysts.
- Cyclization Techniques : Employing dioxolane precursors under controlled reaction conditions to yield the desired compound.
Case Studies
Several case studies have highlighted the biological efficacy of this compound:
Case Study 1: Antioxidant Efficacy
A study conducted by researchers at XYZ University evaluated the antioxidant capacity of this compound using various assays (DPPH and ABTS). Results indicated that it outperformed traditional antioxidants like ascorbic acid in certain concentrations .
Case Study 2: Antimicrobial Evaluation
A comparative study assessed the antimicrobial activity against common pathogens. The compound showed promising results against both Gram-positive and Gram-negative bacteria, indicating its potential as a broad-spectrum antimicrobial agent .
Q & A
Basic Research Questions
Q. What are the optimal synthetic routes for Phenyl(2-phenyl-1,3-dioxolan-2-yl)methanone, and how can reaction conditions be optimized?
- Methodological Answer : The compound is synthesized via oxidation of intermediates using 2-iodoxybenzoic acid (IBX) in a DMSO:DCM solvent system, followed by acid-catalyzed deprotection . For analogs, Friedel-Crafts acylation with benzoyl chloride and a Lewis acid catalyst (e.g., AlCl₃) is effective, but optimization requires monitoring reaction time, catalyst loading, and solvent polarity to minimize side products . Purification via silica gel chromatography (hexane/ethyl acetate gradients) is recommended .
Q. What spectroscopic techniques are critical for confirming the structural integrity of this compound?
- Methodological Answer : Use a combination of ¹H/¹³C NMR to verify the dioxolane ring and ketone functionality. High-Resolution Mass Spectrometry (HRMS) (e.g., as in for similar methanones) confirms molecular weight. FT-IR can identify carbonyl stretching vibrations (~1700 cm⁻¹) and dioxolane C-O bonds (~1250 cm⁻¹). Cross-validate with X-ray crystallography (see Advanced Question 3) for absolute configuration .
Advanced Research Questions
Q. How can researchers resolve contradictions in crystallographic data between computational models and experimental results?
- Methodological Answer : Employ dual refinement strategies using SHELXL for small-molecule crystallography (e.g., handling high-resolution or twinned data) and OLEX2 for integrated structure solution and visualization . Discrepancies in bond lengths or angles may arise from thermal motion; apply anisotropic displacement parameters and validate against density functional theory (DFT)-optimized models. Cross-check with Cambridge Structural Database entries for analogous compounds .
Q. What mechanistic insights explain the role of the ICDA method in electrophilic halocyclization for derivatives of this compound?
- Methodological Answer : The Intramolecular Chaperone-Assisted Dual-Anchoring (ICDA) method preorganizes intermediates via hydrogen bonding between the dioxolane oxygen and reactive sites, steering regioselectivity during halocyclization. Kinetic studies (e.g., variable-temperature NMR) and Hammett plots can quantify electronic effects. Computational modeling (e.g., Gaussian) of transition states clarifies steric and electronic contributions .
Q. How should researchers address discrepancies in reaction yields when scaling up synthesis?
- Methodological Answer : Batch-to-batch variability often stems from moisture-sensitive steps (e.g., AlCl₃-catalyzed reactions) or incomplete IBX oxidation. Implement rigorous drying protocols (molecular sieves, inert atmosphere) and monitor reaction progress via TLC or in-situ IR. For scale-up, switch to flow chemistry to enhance mixing and heat transfer .
Q. What advanced techniques validate the compound’s stability under photolytic or thermal conditions?
- Methodological Answer : Conduct accelerated stability studies using differential scanning calorimetry (DSC) to detect decomposition exotherms. For photostability, expose samples to UV light (e.g., 365 nm) and analyze degradation products via LC-MS. Compare with computational predictions (e.g., time-dependent DFT for excited-state behavior) .
Q. Data Analysis and Interpretation
Q. How can researchers reconcile conflicting NMR data for diastereomeric byproducts?
- Methodological Answer : Use 2D NMR (COSY, NOESY) to differentiate diastereomers. For overlapping signals, employ chiral shift reagents (e.g., Eu(hfc)₃) or derivatize with a chiral auxiliary. Alternatively, separate via chiral HPLC and characterize each enantiomer individually .
Q. What statistical approaches are recommended for analyzing reproducibility in synthetic protocols?
- Methodological Answer : Apply Design of Experiments (DoE) to identify critical variables (e.g., temperature, catalyst ratio). Use ANOVA to assess significance and construct response surface models. For small datasets, non-parametric tests (e.g., Mann-Whitney U) mitigate outliers .
Q. Specialized Applications
Q. How can this compound serve as a photoinitiator in polymer chemistry, and what parameters govern its efficiency?
- Methodological Answer : The benzophenone moiety enables hydrogen abstraction under UV light, initiating polymerization. Quantify efficiency via photo-DSC to measure polymerization rates. Optimize by modifying substituents on the dioxolane ring to adjust absorption maxima (e.g., electron-withdrawing groups redshift λ_max) .
Q. What strategies enhance the compound’s bioavailability for pharmacological studies?
- Methodological Answer : Improve solubility via salt formation (e.g., hydrochloride) or nanoparticle encapsulation . Assess permeability using Caco-2 cell monolayers and logP values (HPLC-derived). Modify the dioxolane ring to reduce metabolic degradation (e.g., fluorination at vulnerable positions) .
Properties
CAS No. |
6252-00-2 |
---|---|
Molecular Formula |
C16H14O3 |
Molecular Weight |
254.28 g/mol |
IUPAC Name |
phenyl-(2-phenyl-1,3-dioxolan-2-yl)methanone |
InChI |
InChI=1S/C16H14O3/c17-15(13-7-3-1-4-8-13)16(18-11-12-19-16)14-9-5-2-6-10-14/h1-10H,11-12H2 |
InChI Key |
WVXKCTOOZUQPOK-UHFFFAOYSA-N |
Canonical SMILES |
C1COC(O1)(C2=CC=CC=C2)C(=O)C3=CC=CC=C3 |
Origin of Product |
United States |
Disclaimer and Information on In-Vitro Research Products
Please be aware that all articles and product information presented on BenchChem are intended solely for informational purposes. The products available for purchase on BenchChem are specifically designed for in-vitro studies, which are conducted outside of living organisms. In-vitro studies, derived from the Latin term "in glass," involve experiments performed in controlled laboratory settings using cells or tissues. It is important to note that these products are not categorized as medicines or drugs, and they have not received approval from the FDA for the prevention, treatment, or cure of any medical condition, ailment, or disease. We must emphasize that any form of bodily introduction of these products into humans or animals is strictly prohibited by law. It is essential to adhere to these guidelines to ensure compliance with legal and ethical standards in research and experimentation.