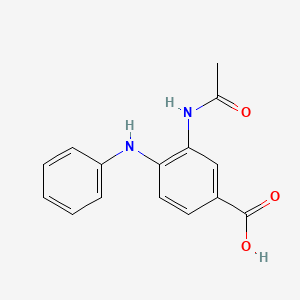
3-(Acetylamino)-4-anilinobenzoic acid
Overview
Description
3-(Acetylamino)-4-anilinobenzoic acid is an organic compound that belongs to the class of acylaminobenzoic acids This compound is characterized by the presence of an acetylamino group and an aniline group attached to a benzoic acid core
Preparation Methods
Synthetic Routes and Reaction Conditions
The synthesis of 3-(Acetylamino)-4-anilinobenzoic acid typically involves the acetylation of 4-anilinobenzoic acid. One common method includes the reaction of 4-anilinobenzoic acid with acetic anhydride in the presence of a catalyst such as pyridine. The reaction is carried out under reflux conditions, and the product is purified by recrystallization.
Industrial Production Methods
On an industrial scale, the production of this compound can be achieved through a similar acetylation process, but with optimized reaction conditions to increase yield and purity. The use of continuous flow reactors and automated systems can enhance the efficiency and scalability of the process.
Chemical Reactions Analysis
Types of Reactions
3-(Acetylamino)-4-anilinobenzoic acid undergoes various chemical reactions, including:
Oxidation: The compound can be oxidized to form corresponding quinones.
Reduction: Reduction reactions can convert the nitro groups to amino groups.
Substitution: Electrophilic substitution reactions can occur on the aromatic ring.
Common Reagents and Conditions
Oxidation: Common oxidizing agents include potassium permanganate and chromium trioxide.
Reduction: Reducing agents such as sodium borohydride or hydrogen gas in the presence of a palladium catalyst are used.
Substitution: Halogenation can be achieved using halogens like chlorine or bromine in the presence of a Lewis acid catalyst.
Major Products
Oxidation: Quinones and other oxidized derivatives.
Reduction: Amino derivatives.
Substitution: Halogenated benzoic acids.
Scientific Research Applications
3-(Acetylamino)-4-anilinobenzoic acid has several applications in scientific research:
Chemistry: Used as an intermediate in the synthesis of dyes and pigments.
Biology: Investigated for its potential as an enzyme inhibitor.
Medicine: Explored for its anti-inflammatory and analgesic properties.
Industry: Utilized in the production of polymers and resins.
Mechanism of Action
The mechanism of action of 3-(Acetylamino)-4-anilinobenzoic acid involves its interaction with specific molecular targets. In medicinal applications, it may inhibit enzymes involved in inflammatory pathways, thereby reducing inflammation and pain. The acetylamino group plays a crucial role in binding to the active site of the target enzyme, blocking its activity.
Comparison with Similar Compounds
Similar Compounds
4-Acetamidobenzoic acid: Similar structure but lacks the aniline group.
N-Acetylanthranilic acid: Contains an acetylamino group but differs in the position of the substituents.
4-Aminobenzoic acid: Similar core structure but lacks the acetylamino group.
Uniqueness
3-(Acetylamino)-4-anilinobenzoic acid is unique due to the presence of both an acetylamino group and an aniline group, which confer distinct chemical properties and reactivity. This combination allows for specific interactions with biological targets and makes it a valuable compound in various applications.
Properties
IUPAC Name |
3-acetamido-4-anilinobenzoic acid | |
---|---|---|
Source | PubChem | |
URL | https://pubchem.ncbi.nlm.nih.gov | |
Description | Data deposited in or computed by PubChem | |
InChI |
InChI=1S/C15H14N2O3/c1-10(18)16-14-9-11(15(19)20)7-8-13(14)17-12-5-3-2-4-6-12/h2-9,17H,1H3,(H,16,18)(H,19,20) | |
Source | PubChem | |
URL | https://pubchem.ncbi.nlm.nih.gov | |
Description | Data deposited in or computed by PubChem | |
InChI Key |
CBMIIHVBLKDQFM-UHFFFAOYSA-N | |
Source | PubChem | |
URL | https://pubchem.ncbi.nlm.nih.gov | |
Description | Data deposited in or computed by PubChem | |
Canonical SMILES |
CC(=O)NC1=C(C=CC(=C1)C(=O)O)NC2=CC=CC=C2 | |
Source | PubChem | |
URL | https://pubchem.ncbi.nlm.nih.gov | |
Description | Data deposited in or computed by PubChem | |
Molecular Formula |
C15H14N2O3 | |
Source | PubChem | |
URL | https://pubchem.ncbi.nlm.nih.gov | |
Description | Data deposited in or computed by PubChem | |
Molecular Weight |
270.28 g/mol | |
Source | PubChem | |
URL | https://pubchem.ncbi.nlm.nih.gov | |
Description | Data deposited in or computed by PubChem | |
Retrosynthesis Analysis
AI-Powered Synthesis Planning: Our tool employs the Template_relevance Pistachio, Template_relevance Bkms_metabolic, Template_relevance Pistachio_ringbreaker, Template_relevance Reaxys, Template_relevance Reaxys_biocatalysis model, leveraging a vast database of chemical reactions to predict feasible synthetic routes.
One-Step Synthesis Focus: Specifically designed for one-step synthesis, it provides concise and direct routes for your target compounds, streamlining the synthesis process.
Accurate Predictions: Utilizing the extensive PISTACHIO, BKMS_METABOLIC, PISTACHIO_RINGBREAKER, REAXYS, REAXYS_BIOCATALYSIS database, our tool offers high-accuracy predictions, reflecting the latest in chemical research and data.
Strategy Settings
Precursor scoring | Relevance Heuristic |
---|---|
Min. plausibility | 0.01 |
Model | Template_relevance |
Template Set | Pistachio/Bkms_metabolic/Pistachio_ringbreaker/Reaxys/Reaxys_biocatalysis |
Top-N result to add to graph | 6 |
Feasible Synthetic Routes
Disclaimer and Information on In-Vitro Research Products
Please be aware that all articles and product information presented on BenchChem are intended solely for informational purposes. The products available for purchase on BenchChem are specifically designed for in-vitro studies, which are conducted outside of living organisms. In-vitro studies, derived from the Latin term "in glass," involve experiments performed in controlled laboratory settings using cells or tissues. It is important to note that these products are not categorized as medicines or drugs, and they have not received approval from the FDA for the prevention, treatment, or cure of any medical condition, ailment, or disease. We must emphasize that any form of bodily introduction of these products into humans or animals is strictly prohibited by law. It is essential to adhere to these guidelines to ensure compliance with legal and ethical standards in research and experimentation.