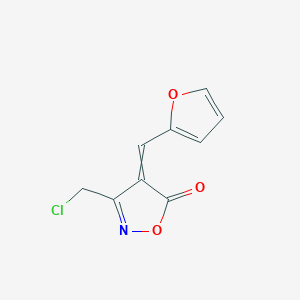
3-(Chloromethyl)-4-(furan-2-ylmethylidene)-1,2-oxazol-5-one
Overview
Description
3-(Chloromethyl)-4-(furan-2-ylmethylidene)-1,2-oxazol-5-one is a heterocyclic compound that features a unique combination of functional groups, including a chloromethyl group, a furan ring, and an oxazole ring
Preparation Methods
Synthetic Routes and Reaction Conditions
The synthesis of 3-(Chloromethyl)-4-(furan-2-ylmethylidene)-1,2-oxazol-5-one typically involves the reaction of furan-2-carbaldehyde with chloromethyl ketone in the presence of a base. The reaction proceeds through a condensation mechanism, forming the oxazole ring as a key intermediate. The reaction conditions often include the use of solvents such as ethanol or acetonitrile and a base like sodium hydroxide or potassium carbonate.
Industrial Production Methods
Industrial production of this compound may involve similar synthetic routes but on a larger scale. The process would be optimized for yield and purity, often involving continuous flow reactors and automated systems to ensure consistent production. The choice of solvents and reagents would be influenced by factors such as cost, availability, and environmental impact.
Chemical Reactions Analysis
Types of Reactions
3-(Chloromethyl)-4-(furan-2-ylmethylidene)-1,2-oxazol-5-one can undergo various chemical reactions, including:
Oxidation: The furan ring can be oxidized to form furanones.
Reduction: The oxazole ring can be reduced to form corresponding amines.
Substitution: The chloromethyl group can undergo nucleophilic substitution reactions to form a variety of derivatives.
Common Reagents and Conditions
Oxidation: Common oxidizing agents include potassium permanganate and chromium trioxide.
Reduction: Reducing agents such as lithium aluminum hydride or sodium borohydride are typically used.
Substitution: Nucleophiles like amines, thiols, and alcohols can react with the chloromethyl group under mild conditions.
Major Products
The major products formed from these reactions depend on the specific reagents and conditions used. For example, oxidation of the furan ring can yield furanones, while nucleophilic substitution of the chloromethyl group can produce a wide range of substituted oxazoles.
Scientific Research Applications
3-(Chloromethyl)-4-(furan-2-ylmethylidene)-1,2-oxazol-5-one has several applications in scientific research:
Chemistry: It serves as a building block for the synthesis of more complex molecules and can be used in the development of new synthetic methodologies.
Biology: The compound’s unique structure makes it a candidate for studying enzyme interactions and biological pathways.
Industry: It can be used in the production of advanced materials, such as polymers and coatings, due to its reactive functional groups.
Mechanism of Action
The mechanism by which 3-(Chloromethyl)-4-(furan-2-ylmethylidene)-1,2-oxazol-5-one exerts its effects depends on the specific application. In medicinal chemistry, it may interact with enzymes or receptors through its functional groups, forming covalent or non-covalent bonds. The furan and oxazole rings can participate in π-π stacking interactions, while the chloromethyl group can form covalent bonds with nucleophilic sites on proteins or other biomolecules.
Comparison with Similar Compounds
Similar Compounds
3-(Chloromethyl)-4-(phenylmethylidene)-1,2-oxazol-5-one: Similar structure but with a phenyl group instead of a furan ring.
3-(Bromomethyl)-4-(furan-2-ylmethylidene)-1,2-oxazol-5-one: Similar structure but with a bromomethyl group instead of a chloromethyl group.
4-(Furan-2-ylmethylidene)-1,2-oxazol-5-one: Lacks the chloromethyl group.
Uniqueness
3-(Chloromethyl)-4-(furan-2-ylmethylidene)-1,2-oxazol-5-one is unique due to the presence of both a chloromethyl group and a furan ring, which confer distinct reactivity and potential for diverse applications. The combination of these functional groups allows for a wide range of chemical transformations and interactions, making it a versatile compound in various fields of research.
Properties
IUPAC Name |
3-(chloromethyl)-4-(furan-2-ylmethylidene)-1,2-oxazol-5-one | |
---|---|---|
Source | PubChem | |
URL | https://pubchem.ncbi.nlm.nih.gov | |
Description | Data deposited in or computed by PubChem | |
InChI |
InChI=1S/C9H6ClNO3/c10-5-8-7(9(12)14-11-8)4-6-2-1-3-13-6/h1-4H,5H2 | |
Source | PubChem | |
URL | https://pubchem.ncbi.nlm.nih.gov | |
Description | Data deposited in or computed by PubChem | |
InChI Key |
VYJBJWAFWOFHSB-UHFFFAOYSA-N | |
Source | PubChem | |
URL | https://pubchem.ncbi.nlm.nih.gov | |
Description | Data deposited in or computed by PubChem | |
Canonical SMILES |
C1=COC(=C1)C=C2C(=NOC2=O)CCl | |
Source | PubChem | |
URL | https://pubchem.ncbi.nlm.nih.gov | |
Description | Data deposited in or computed by PubChem | |
Molecular Formula |
C9H6ClNO3 | |
Source | PubChem | |
URL | https://pubchem.ncbi.nlm.nih.gov | |
Description | Data deposited in or computed by PubChem | |
Molecular Weight |
211.60 g/mol | |
Source | PubChem | |
URL | https://pubchem.ncbi.nlm.nih.gov | |
Description | Data deposited in or computed by PubChem | |
Retrosynthesis Analysis
AI-Powered Synthesis Planning: Our tool employs the Template_relevance Pistachio, Template_relevance Bkms_metabolic, Template_relevance Pistachio_ringbreaker, Template_relevance Reaxys, Template_relevance Reaxys_biocatalysis model, leveraging a vast database of chemical reactions to predict feasible synthetic routes.
One-Step Synthesis Focus: Specifically designed for one-step synthesis, it provides concise and direct routes for your target compounds, streamlining the synthesis process.
Accurate Predictions: Utilizing the extensive PISTACHIO, BKMS_METABOLIC, PISTACHIO_RINGBREAKER, REAXYS, REAXYS_BIOCATALYSIS database, our tool offers high-accuracy predictions, reflecting the latest in chemical research and data.
Strategy Settings
Precursor scoring | Relevance Heuristic |
---|---|
Min. plausibility | 0.01 |
Model | Template_relevance |
Template Set | Pistachio/Bkms_metabolic/Pistachio_ringbreaker/Reaxys/Reaxys_biocatalysis |
Top-N result to add to graph | 6 |
Feasible Synthetic Routes
Disclaimer and Information on In-Vitro Research Products
Please be aware that all articles and product information presented on BenchChem are intended solely for informational purposes. The products available for purchase on BenchChem are specifically designed for in-vitro studies, which are conducted outside of living organisms. In-vitro studies, derived from the Latin term "in glass," involve experiments performed in controlled laboratory settings using cells or tissues. It is important to note that these products are not categorized as medicines or drugs, and they have not received approval from the FDA for the prevention, treatment, or cure of any medical condition, ailment, or disease. We must emphasize that any form of bodily introduction of these products into humans or animals is strictly prohibited by law. It is essential to adhere to these guidelines to ensure compliance with legal and ethical standards in research and experimentation.