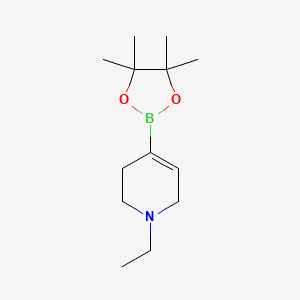
1-Ethyl-4-(4,4,5,5-tetramethyl-1,3,2-dioxaborolan-2-YL)-1,2,3,6-tetrahydropyridine
Overview
Description
Synthesis Analysis
The synthesis of this compound is not explicitly mentioned in the sources I found .
Chemical Reactions Analysis
Information about the chemical reactions involving this compound is not available in the sources I found .
Physical And Chemical Properties Analysis
This compound is a liquid at 20 degrees Celsius . It should be stored under inert gas as it is air sensitive . The molecular weight of the compound is 222.10 g/mol .
Scientific Research Applications
Synthesis and Structural Analysis
1-Ethyl-4-(4,4,5,5-tetramethyl-1,3,2-dioxaborolan-2-yl)-1,2,3,6-tetrahydropyridine and similar compounds are primarily used as intermediates in chemical synthesis. For example, the synthesis and crystal structure of related boric acid ester intermediates have been studied, confirming their structures through various spectroscopic methods and X-ray diffraction. These compounds were synthesized via a three-step substitution reaction. Conformational and crystallographic analyses were conducted, and the structures were further validated through density functional theory (DFT) calculations (Huang et al., 2021).
Application in Material Science
These compounds are used in material science, particularly in the synthesis of electron transport materials. For instance, an efficient synthesis process was developed for an electron transport material and its key intermediate, using these compounds. This development opens doors for further applications in the synthesis of other materials in this field (Xiangdong et al., 2017).
Use in Fluorescence Probes and Sensors
1-Ethyl-4-(4,4,5,5-tetramethyl-1,3,2-dioxaborolan-2-yl)-1,2,3,6-tetrahydropyridine derivatives have also found application in the development of fluorescence probes and sensors. For example, a series of boronate ester fluorescence probes were synthesized for the detection of hydrogen peroxide, showcasing the versatility of these compounds in sensor applications (Lampard et al., 2018).
Catalysis and Organic Reactions
These compounds are instrumental in catalysis and organic reactions. For instance, they have been used in palladium-catalyzed reactions, which are crucial in organic synthesis. The efficiency of these reactions in various chemical processes highlights the importance of these compounds in synthetic chemistry (Zhu et al., 2003).
Antifungal and Antibacterial Properties
Additionally, derivatives of these compounds have shown significant antifungal and antibacterial activities. This suggests potential applications in the development of new antimicrobial agents, expanding their use beyond purely chemical synthesis (Irving et al., 2003).
Mechanism of Action
Safety and Hazards
This compound can cause skin irritation and serious eye irritation . Therefore, it is recommended to wear protective gloves, eye protection, and face protection when handling this compound . If it comes into contact with the skin, wash with plenty of water . If eye irritation persists, seek medical advice or attention .
properties
IUPAC Name |
1-ethyl-4-(4,4,5,5-tetramethyl-1,3,2-dioxaborolan-2-yl)-3,6-dihydro-2H-pyridine | |
---|---|---|
Source | PubChem | |
URL | https://pubchem.ncbi.nlm.nih.gov | |
Description | Data deposited in or computed by PubChem | |
InChI |
InChI=1S/C13H24BNO2/c1-6-15-9-7-11(8-10-15)14-16-12(2,3)13(4,5)17-14/h7H,6,8-10H2,1-5H3 | |
Source | PubChem | |
URL | https://pubchem.ncbi.nlm.nih.gov | |
Description | Data deposited in or computed by PubChem | |
InChI Key |
FWEAXLMLFSWZEI-UHFFFAOYSA-N | |
Source | PubChem | |
URL | https://pubchem.ncbi.nlm.nih.gov | |
Description | Data deposited in or computed by PubChem | |
Canonical SMILES |
B1(OC(C(O1)(C)C)(C)C)C2=CCN(CC2)CC | |
Source | PubChem | |
URL | https://pubchem.ncbi.nlm.nih.gov | |
Description | Data deposited in or computed by PubChem | |
Molecular Formula |
C13H24BNO2 | |
Source | PubChem | |
URL | https://pubchem.ncbi.nlm.nih.gov | |
Description | Data deposited in or computed by PubChem | |
Molecular Weight |
237.15 g/mol | |
Source | PubChem | |
URL | https://pubchem.ncbi.nlm.nih.gov | |
Description | Data deposited in or computed by PubChem | |
Product Name |
1-Ethyl-4-(4,4,5,5-tetramethyl-1,3,2-dioxaborolan-2-YL)-1,2,3,6-tetrahydropyridine |
Retrosynthesis Analysis
AI-Powered Synthesis Planning: Our tool employs the Template_relevance Pistachio, Template_relevance Bkms_metabolic, Template_relevance Pistachio_ringbreaker, Template_relevance Reaxys, Template_relevance Reaxys_biocatalysis model, leveraging a vast database of chemical reactions to predict feasible synthetic routes.
One-Step Synthesis Focus: Specifically designed for one-step synthesis, it provides concise and direct routes for your target compounds, streamlining the synthesis process.
Accurate Predictions: Utilizing the extensive PISTACHIO, BKMS_METABOLIC, PISTACHIO_RINGBREAKER, REAXYS, REAXYS_BIOCATALYSIS database, our tool offers high-accuracy predictions, reflecting the latest in chemical research and data.
Strategy Settings
Precursor scoring | Relevance Heuristic |
---|---|
Min. plausibility | 0.01 |
Model | Template_relevance |
Template Set | Pistachio/Bkms_metabolic/Pistachio_ringbreaker/Reaxys/Reaxys_biocatalysis |
Top-N result to add to graph | 6 |
Feasible Synthetic Routes
Disclaimer and Information on In-Vitro Research Products
Please be aware that all articles and product information presented on BenchChem are intended solely for informational purposes. The products available for purchase on BenchChem are specifically designed for in-vitro studies, which are conducted outside of living organisms. In-vitro studies, derived from the Latin term "in glass," involve experiments performed in controlled laboratory settings using cells or tissues. It is important to note that these products are not categorized as medicines or drugs, and they have not received approval from the FDA for the prevention, treatment, or cure of any medical condition, ailment, or disease. We must emphasize that any form of bodily introduction of these products into humans or animals is strictly prohibited by law. It is essential to adhere to these guidelines to ensure compliance with legal and ethical standards in research and experimentation.