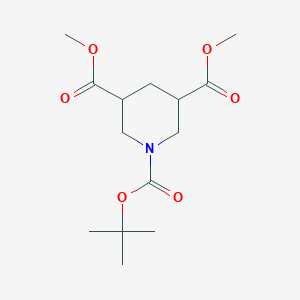
1-Tert-butyl 3,5-dimethyl piperidine-1,3,5-tricarboxylate
Overview
Description
1-Tert-butyl 3,5-dimethyl piperidine-1,3,5-tricarboxylate (CAS: 595555-70-7) is a piperidine-based tricarboxylate ester featuring tert-butyl and methyl ester groups. Its synthesis involves catalytic hydrogenation and acid-mediated esterification steps, yielding a crystalline powder with a purity of 95% . Key structural features include a six-membered piperidine ring and three carboxylate groups, which confer steric bulk and modulate reactivity. Nuclear magnetic resonance (NMR) data (e.g., δ 1.46 ppm for tert-butyl protons and δ 3.64–3.81 ppm for methyl esters) confirm its stereochemistry . The compound is pivotal in medicinal chemistry, particularly as a scaffold in RORγ inhibitors, where its 3,5-dimethyl piperidine moiety enhances nanomolar potency .
Preparation Methods
Preparation Methods
General Synthetic Strategy
The synthesis of 1-tert-butyl 3,5-dimethyl piperidine-1,3,5-tricarboxylate generally involves:
- Starting from piperidine derivatives bearing carboxylate groups.
- Selective protection of carboxyl groups as tert-butyl esters.
- Introduction of methyl groups at the 3 and 5 positions.
- Use of bases and controlled reaction conditions to facilitate esterification and functional group transformations.
Preparation from Di-tert-butyl Dicarbonate and Dimethyl Piperidine-3,5-dicarboxylate
One documented method involves the reaction of di-tert-butyl dicarbonate (Boc anhydride) with dimethyl piperidine-3,5-dicarboxylate in the presence of sodium bicarbonate in a tetrahydrofuran (THF)/water mixture at ambient temperature (~18 °C) for about 2 hours. This reaction selectively introduces the tert-butyl protecting group at the nitrogen atom of the piperidine ring, yielding the desired triester compound.
Reagents/Conditions | Details |
---|---|
Starting material | Dimethyl piperidine-3,5-dicarboxylate (6.00 g, 29.82 mmol) |
Protecting agent | Di-tert-butyl dicarbonate (Boc anhydride) (excess) |
Base | Sodium bicarbonate (NaHCO3) (10.02 g, 119.27 mmol) |
Solvent | Tetrahydrofuran (20 mL) and water (5 mL) |
Temperature | 18 °C |
Reaction time | 2 hours |
Yield | 7.2 g (approximate) |
This method provides efficient tert-butyl protection of the nitrogen and carboxyl groups, facilitating further synthetic manipulations.
Hydrolysis and Subsequent Acidification
In a related process, the triester compound can be subjected to hydrolysis under basic conditions followed by acidification to obtain related carboxylic acid derivatives:
- The triester is dissolved in methanol.
- Sodium hydroxide aqueous solution is added dropwise at room temperature (10–35 °C).
- The mixture is stirred for 14 hours.
- Methanol is removed under reduced pressure.
- The aqueous phase is neutralized to pH 2 with hydrochloric acid.
- The product is extracted with ethyl acetate, dried, and concentrated to yield the acid form.
This hydrolysis step is crucial for modifying ester groups and obtaining intermediates for further functionalization or purification.
Use of Metal Bases for Deprotection and Functionalization
Another important aspect in the preparation involves the use of metal bases such as alkali metal hydroxides (e.g., sodium hydroxide, potassium hydroxide), metal alkoxides (e.g., sodium methoxide, potassium tert-butoxide), metal amides, or metal carbonates to facilitate deprotection or substitution reactions on related piperidine carboxylate derivatives.
- These bases are used in 1–10 molar equivalents relative to the substrate.
- Solvents include water, tetrahydrofuran, ethers, alcohols, and polar aprotic solvents like dimethyl sulfoxide or N,N-dimethylformamide.
- Reaction temperatures range from 0 to 160 °C, typically 50–120 °C.
- Reaction times vary from 15 minutes to 12 hours depending on conditions.
- The reaction progress is monitored by chromatographic methods such as GC or HPLC.
This approach is particularly useful for selective deprotection of alkoxycarbonylamino groups on piperidine rings to yield tert-butyl 3-aminopiperidine-1-carboxylate intermediates, which can be further elaborated.
Analytical and Purification Techniques
- Chromatography: Silica gel column chromatography and preparative HPLC are commonly used to purify intermediates and the final compound.
- Spectroscopic Confirmation: 1H-NMR and ESI-MS are employed to confirm the structure and purity of synthesized compounds.
- Crystallization: Post-reaction mixtures are often subjected to crystallization or extraction to isolate pure products.
- Reaction Monitoring: Gas chromatography (GC) and high-performance liquid chromatography (HPLC) are standard for monitoring reaction progress.
Summary Table of Key Preparation Parameters
Step | Reagents/Conditions | Temperature (°C) | Time | Yield/Notes |
---|---|---|---|---|
Boc Protection | Di-tert-butyl dicarbonate, NaHCO3, THF/H2O | 18 | 2 h | ~7.2 g product from 6 g starting |
Hydrolysis & Acidification | NaOH in MeOH, then HCl to pH 2 | 10–35 | 14 h | Acid form isolated by extraction |
Deprotection with Metal Bases | Metal hydroxides/alkoxides, various solvents | 0–160 (prefer 50–120) | 15 min–12 h | Reaction monitored by GC/HPLC |
Purification | Silica gel chromatography, HPLC | Ambient | Variable | Confirmed by NMR, MS |
Research Findings and Practical Considerations
- The choice of base and solvent critically influences the yield and selectivity of tert-butyl ester formation and deprotection steps.
- Sodium bicarbonate in aqueous-organic solvent mixtures is effective for mild Boc protection of piperidine nitrogen.
- Longer reaction times and moderate temperatures favor complete conversion in hydrolysis steps without decomposition.
- Use of metal alkoxides and hydroxides allows for selective deprotection and functional group transformations on piperidine derivatives.
- Monitoring by chromatographic techniques ensures control over reaction progress and purity.
- Final compounds are stable under standard storage conditions when isolated as tert-butyl esters.
This comprehensive analysis of the preparation methods for this compound integrates data from patent literature and chemical synthesis reports, providing a reliable and authoritative guide for researchers and chemists working with this compound.
Chemical Reactions Analysis
1-Tert-butyl 3,5-dimethyl piperidine-1,3,5-tricarboxylate undergoes various chemical reactions, including:
Oxidation: This compound can be oxidized using common oxidizing agents such as potassium permanganate or chromium trioxide, leading to the formation of oxidized derivatives.
Reduction: Reduction reactions can be carried out using reducing agents like lithium aluminum hydride, resulting in the formation of reduced piperidine derivatives.
Substitution: The compound can undergo substitution reactions where one or more substituents on the piperidine ring are replaced by other functional groups.
The major products formed from these reactions depend on the specific reagents and conditions used. For example, oxidation may yield carboxylic acids, while reduction may produce alcohols or amines.
Scientific Research Applications
Medicinal Chemistry
1-Tert-butyl 3,5-dimethyl piperidine-1,3,5-tricarboxylate is primarily investigated for its potential as a pharmaceutical intermediate. Its structural features allow it to serve as a building block for various bioactive compounds.
Case Study: Synthesis of Piperidine Derivatives
Research has demonstrated that derivatives of this compound can exhibit significant activity against certain types of cancer cells. For instance, modifications to the piperidine ring have led to compounds with enhanced anti-tumor properties .
Organic Synthesis
The compound is utilized in the synthesis of complex organic molecules due to its ability to undergo various chemical reactions such as esterification and amide formation.
Data Table: Common Reactions Involving this compound
Reaction Type | Description | Reference |
---|---|---|
Esterification | Reacts with alcohols to form esters | |
Amide Formation | Forms amides upon reaction with amines | |
Alkylation | Can be alkylated to introduce longer carbon chains |
Agrochemicals
This compound has also been explored for its potential use in agrochemicals. Its structure allows it to interact with biological systems in ways that could lead to the development of new pesticides or herbicides.
Case Study: Herbicidal Activity
In preliminary studies, derivatives of this compound showed promising herbicidal activity against specific weed species, suggesting potential applications in agricultural practices .
Mechanism of Action
The mechanism of action of 1-Tert-butyl 3,5-dimethyl piperidine-1,3,5-tricarboxylate involves its interaction with molecular targets such as enzymes and receptors. The compound’s piperidine ring structure allows it to bind to specific sites on these targets, modulating their activity. The tert-butyl and dimethyl groups contribute to the compound’s binding affinity and specificity, while the carboxylate groups enhance its solubility and reactivity .
Comparison with Similar Compounds
Comparison with Structurally Similar Compounds
Piperidine and Pyridine Tricarboxylates
- Key Differences :
- The target compound’s piperidine core provides conformational rigidity compared to dihydropyridine derivatives, enhancing receptor binding .
- Chloromethyl groups in the dihydropyridine analog increase electrophilicity, favoring nucleophilic substitutions absent in the tert-butyl/methyl-protected target compound .
Aromatic Tricarboxylates in Coordination Polymers
- Key Differences :
- Aromatic tricarboxylates like Cu-BTC form metal-organic frameworks (MOFs) with applications in gas storage, contrasting with the piperidine derivative’s role in drug design .
- Chloromethyl substituents in benzene tricarboxylates enable polymer functionalization, unlike the hydrolytically stable tert-butyl groups in the target compound .
Alicyclic Tricarboxylates
- Key Differences :
- The cyclohexane derivative’s pentacarboxylate structure increases steric hindrance, reducing reactivity compared to the piperidine tricarboxylate’s three ester groups .
Biological Activity
1-Tert-butyl 3,5-dimethyl piperidine-1,3,5-tricarboxylate (CAS No. 595555-70-7) is a synthetic compound that has garnered attention in the field of medicinal chemistry due to its potential biological activities. This article reviews its synthesis, biological properties, and relevant research findings.
Chemical Structure and Properties
This compound has the molecular formula and a molecular weight of approximately 301.336 g/mol. The compound features a piperidine ring substituted with three carboxylate groups and tert-butyl and dimethyl groups at specific positions, contributing to its unique chemical behavior and biological activity .
Synthesis
The synthesis of this compound involves several steps:
- Dissolving a precursor in ethanol.
- Adding triethylamine and di-tert-butyl bicarbonate under controlled conditions.
- Extracting the product using ethyl acetate and purifying it via silica gel column chromatography .
Cytotoxicity and Pharmacological Effects
Preliminary evaluations suggest that compounds with similar structures may exhibit cytotoxic effects against cancer cell lines. The presence of multiple carboxylate groups can enhance interactions with biological targets, potentially leading to apoptosis in cancer cells. However, detailed studies specifically focusing on the cytotoxicity of this compound are necessary to confirm these effects.
Case Studies and Research Findings
Recent studies have focused on the pharmacological properties of various piperidine derivatives:
- A study explored the synthesis and biological evaluation of piperidinothiosemicarbazones, which showed promising results against M. tuberculosis strains . This highlights the importance of structural modifications in enhancing biological activity.
- Another investigation into related compounds indicated that structural variations significantly affect their antimicrobial potency .
Data Table: Biological Activity Comparison
Compound Name | MIC (µg/mL) | Activity Description |
---|---|---|
Compound DMK-20 (piperidinothiosemicarbazone) | 2 | Strong activity against M. tuberculosis |
Compound DMK-16 (piperidinothiosemicarbazone) | 4 | Moderate activity against resistant strains |
This compound | TBD | Potential based on structural similarity |
Q & A
Basic Research Questions
Q. What are the recommended synthetic routes for 1-Tert-butyl 3,5-dimethyl piperidine-1,3,5-tricarboxylate, and how can purity be optimized?
The compound is typically synthesized via multi-step esterification and protection/deprotection strategies. A common approach involves reacting piperidine derivatives with tert-butyl and methyl carboxylating agents under anhydrous conditions. Key steps include:
- Stepwise esterification : Sequential introduction of tert-butyl and methyl groups to avoid steric hindrance.
- Purification : Recrystallization or column chromatography using silica gel, with purity confirmed by melting point analysis (e.g., 84–86.5°C for analogous tert-butyl esters) .
- Characterization : NMR (¹H/¹³C) and IR spectroscopy to verify functional groups, complemented by mass spectrometry for molecular weight confirmation.
Q. Which spectroscopic techniques are critical for characterizing this compound, and how are spectral contradictions resolved?
- Primary techniques : ¹H NMR (to identify tert-butyl protons at ~1.4 ppm and methyl ester protons at ~3.7 ppm) and ¹³C NMR (carbonyl signals near 170 ppm).
- Contradiction resolution : Discrepancies between calculated and observed spectra may arise from conformational flexibility. Cross-validation via X-ray crystallography (as demonstrated for structurally similar piperidine carboxylates) can resolve ambiguities .
Q. What safety protocols are essential when handling this compound in laboratory settings?
- PPE : Respiratory protection (N95 masks), nitrile gloves, and chemical goggles .
- Ventilation : Use fume hoods during synthesis to mitigate inhalation risks.
- Emergency measures : Immediate eye washing and medical consultation if exposed, as per ECHA guidelines for pyridine derivatives .
Advanced Research Questions
Q. How can computational methods elucidate the reaction mechanisms of this compound in multi-step syntheses?
- Reaction path search : Quantum chemical calculations (e.g., density functional theory) model transition states and intermediates. For example, ICReDD’s approach combines computational screening with experimental validation to identify energetically favorable pathways .
- Optimization : Machine learning algorithms analyze reaction parameters (e.g., solvent polarity, temperature) to predict optimal yields .
Q. What experimental design frameworks are suitable for studying its reactivity in complex reaction systems?
- Factorial design : Systematic variation of factors like catalyst loading, temperature, and solvent polarity to identify dominant variables. For instance, a 2³ factorial design could optimize esterification efficiency .
- Process simulation : Software like Aspen Plus models scale-up challenges, such as heat transfer in continuous-flow reactors .
Q. How can researchers address contradictions between experimental data and theoretical predictions?
- Case study : If observed reaction rates deviate from computational predictions, conduct sensitivity analysis on input parameters (e.g., steric effects of tert-butyl groups).
- Data integration : Cross-reference computational results with high-resolution mass spectrometry (HRMS) and X-ray crystallography to validate structural hypotheses .
Q. What methodologies are effective for studying its stability under varying pH and temperature conditions?
- Degradation kinetics : Accelerated stability studies (40°C/75% RH) with HPLC monitoring.
- Mechanistic insights : pH-dependent hydrolysis of ester groups can be modeled using Arrhenius equations, with degradation products identified via LC-MS .
Q. How can molecular dynamics simulations enhance understanding of its interactions in catalytic systems?
- Binding affinity studies : Simulations predict interactions with enzymes or metal catalysts, focusing on the tert-butyl group’s steric influence.
- Validation : Compare simulated binding energies with experimental kinetic data (e.g., turnover frequencies) .
Q. What strategies mitigate challenges in scaling up its synthesis from lab to pilot plant?
Properties
IUPAC Name |
1-O-tert-butyl 3-O,5-O-dimethyl piperidine-1,3,5-tricarboxylate | |
---|---|---|
Source | PubChem | |
URL | https://pubchem.ncbi.nlm.nih.gov | |
Description | Data deposited in or computed by PubChem | |
InChI |
InChI=1S/C14H23NO6/c1-14(2,3)21-13(18)15-7-9(11(16)19-4)6-10(8-15)12(17)20-5/h9-10H,6-8H2,1-5H3 | |
Source | PubChem | |
URL | https://pubchem.ncbi.nlm.nih.gov | |
Description | Data deposited in or computed by PubChem | |
InChI Key |
MIZBOYNXOLJHQY-UHFFFAOYSA-N | |
Source | PubChem | |
URL | https://pubchem.ncbi.nlm.nih.gov | |
Description | Data deposited in or computed by PubChem | |
Canonical SMILES |
CC(C)(C)OC(=O)N1CC(CC(C1)C(=O)OC)C(=O)OC | |
Source | PubChem | |
URL | https://pubchem.ncbi.nlm.nih.gov | |
Description | Data deposited in or computed by PubChem | |
Molecular Formula |
C14H23NO6 | |
Source | PubChem | |
URL | https://pubchem.ncbi.nlm.nih.gov | |
Description | Data deposited in or computed by PubChem | |
Molecular Weight |
301.34 g/mol | |
Source | PubChem | |
URL | https://pubchem.ncbi.nlm.nih.gov | |
Description | Data deposited in or computed by PubChem | |
Retrosynthesis Analysis
AI-Powered Synthesis Planning: Our tool employs the Template_relevance Pistachio, Template_relevance Bkms_metabolic, Template_relevance Pistachio_ringbreaker, Template_relevance Reaxys, Template_relevance Reaxys_biocatalysis model, leveraging a vast database of chemical reactions to predict feasible synthetic routes.
One-Step Synthesis Focus: Specifically designed for one-step synthesis, it provides concise and direct routes for your target compounds, streamlining the synthesis process.
Accurate Predictions: Utilizing the extensive PISTACHIO, BKMS_METABOLIC, PISTACHIO_RINGBREAKER, REAXYS, REAXYS_BIOCATALYSIS database, our tool offers high-accuracy predictions, reflecting the latest in chemical research and data.
Strategy Settings
Precursor scoring | Relevance Heuristic |
---|---|
Min. plausibility | 0.01 |
Model | Template_relevance |
Template Set | Pistachio/Bkms_metabolic/Pistachio_ringbreaker/Reaxys/Reaxys_biocatalysis |
Top-N result to add to graph | 6 |
Feasible Synthetic Routes
Disclaimer and Information on In-Vitro Research Products
Please be aware that all articles and product information presented on BenchChem are intended solely for informational purposes. The products available for purchase on BenchChem are specifically designed for in-vitro studies, which are conducted outside of living organisms. In-vitro studies, derived from the Latin term "in glass," involve experiments performed in controlled laboratory settings using cells or tissues. It is important to note that these products are not categorized as medicines or drugs, and they have not received approval from the FDA for the prevention, treatment, or cure of any medical condition, ailment, or disease. We must emphasize that any form of bodily introduction of these products into humans or animals is strictly prohibited by law. It is essential to adhere to these guidelines to ensure compliance with legal and ethical standards in research and experimentation.