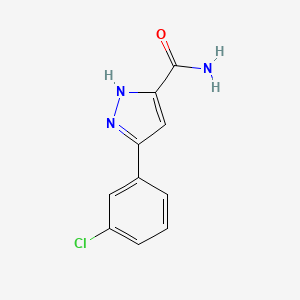
3-(3-Chlorophenyl)-1H-pyrazole-5-carboxamide
Overview
Description
3-(3-Chlorophenyl)-1H-pyrazole-5-carboxamide is a chemical compound that belongs to the class of pyrazoles Pyrazoles are five-membered heterocyclic compounds containing two adjacent nitrogen atoms This particular compound is characterized by the presence of a chlorophenyl group at the 3-position and a carboxamide group at the 5-position of the pyrazole ring
Preparation Methods
Synthetic Routes and Reaction Conditions
The synthesis of 3-(3-Chlorophenyl)-1H-pyrazole-5-carboxamide typically involves the reaction of 3-chlorobenzoyl chloride with hydrazine hydrate to form 3-(3-chlorophenyl)hydrazine. This intermediate is then cyclized with ethyl acetoacetate under basic conditions to yield the desired pyrazole compound. The reaction conditions often include the use of solvents such as ethanol or methanol and catalysts like sodium ethoxide.
Industrial Production Methods
Industrial production methods for this compound may involve similar synthetic routes but are optimized for large-scale production. This includes the use of continuous flow reactors to ensure consistent product quality and yield. Additionally, industrial methods may incorporate more efficient purification techniques such as crystallization or chromatography to obtain high-purity products.
Chemical Reactions Analysis
Types of Reactions
3-(3-Chlorophenyl)-1H-pyrazole-5-carboxamide undergoes various chemical reactions, including:
Oxidation: The compound can be oxidized using reagents like potassium permanganate or hydrogen peroxide.
Reduction: Reduction reactions can be carried out using agents such as sodium borohydride or lithium aluminum hydride.
Substitution: The chlorophenyl group can undergo nucleophilic substitution reactions with reagents like sodium methoxide or potassium tert-butoxide.
Common Reagents and Conditions
Oxidation: Potassium permanganate in acidic or neutral conditions.
Reduction: Sodium borohydride in methanol or ethanol.
Substitution: Sodium methoxide in methanol or potassium tert-butoxide in tert-butanol.
Major Products Formed
Oxidation: Formation of carboxylic acids or ketones.
Reduction: Formation of amines or alcohols.
Substitution: Formation of substituted pyrazoles with various functional groups.
Scientific Research Applications
3-(3-Chlorophenyl)-1H-pyrazole-5-carboxamide has several scientific research applications:
Chemistry: Used as a building block in the synthesis of more complex molecules.
Biology: Investigated for its potential as an enzyme inhibitor or receptor modulator.
Medicine: Explored for its potential therapeutic effects, including anti-inflammatory and analgesic properties.
Industry: Utilized in the development of new materials with specific properties, such as polymers or coatings.
Mechanism of Action
The mechanism of action of 3-(3-Chlorophenyl)-1H-pyrazole-5-carboxamide involves its interaction with specific molecular targets. It may act as an inhibitor of certain enzymes or receptors, thereby modulating biological pathways. The exact molecular targets and pathways can vary depending on the specific application and context of use.
Comparison with Similar Compounds
Similar Compounds
- 3-(2-Chlorophenyl)-1H-pyrazole-5-carboxamide
- 3-(4-Chlorophenyl)-1H-pyrazole-5-carboxamide
- 3-(3-Bromophenyl)-1H-pyrazole-5-carboxamide
Uniqueness
3-(3-Chlorophenyl)-1H-pyrazole-5-carboxamide is unique due to the specific positioning of the chlorophenyl group, which can influence its reactivity and interaction with biological targets. This positional specificity can result in distinct pharmacological or material properties compared to its analogs.
Properties
IUPAC Name |
3-(3-chlorophenyl)-1H-pyrazole-5-carboxamide | |
---|---|---|
Source | PubChem | |
URL | https://pubchem.ncbi.nlm.nih.gov | |
Description | Data deposited in or computed by PubChem | |
InChI |
InChI=1S/C10H8ClN3O/c11-7-3-1-2-6(4-7)8-5-9(10(12)15)14-13-8/h1-5H,(H2,12,15)(H,13,14) | |
Source | PubChem | |
URL | https://pubchem.ncbi.nlm.nih.gov | |
Description | Data deposited in or computed by PubChem | |
InChI Key |
GRLDUFMWSZYNSH-UHFFFAOYSA-N | |
Source | PubChem | |
URL | https://pubchem.ncbi.nlm.nih.gov | |
Description | Data deposited in or computed by PubChem | |
Canonical SMILES |
C1=CC(=CC(=C1)Cl)C2=NNC(=C2)C(=O)N | |
Source | PubChem | |
URL | https://pubchem.ncbi.nlm.nih.gov | |
Description | Data deposited in or computed by PubChem | |
Molecular Formula |
C10H8ClN3O | |
Source | PubChem | |
URL | https://pubchem.ncbi.nlm.nih.gov | |
Description | Data deposited in or computed by PubChem | |
Molecular Weight |
221.64 g/mol | |
Source | PubChem | |
URL | https://pubchem.ncbi.nlm.nih.gov | |
Description | Data deposited in or computed by PubChem | |
Retrosynthesis Analysis
AI-Powered Synthesis Planning: Our tool employs the Template_relevance Pistachio, Template_relevance Bkms_metabolic, Template_relevance Pistachio_ringbreaker, Template_relevance Reaxys, Template_relevance Reaxys_biocatalysis model, leveraging a vast database of chemical reactions to predict feasible synthetic routes.
One-Step Synthesis Focus: Specifically designed for one-step synthesis, it provides concise and direct routes for your target compounds, streamlining the synthesis process.
Accurate Predictions: Utilizing the extensive PISTACHIO, BKMS_METABOLIC, PISTACHIO_RINGBREAKER, REAXYS, REAXYS_BIOCATALYSIS database, our tool offers high-accuracy predictions, reflecting the latest in chemical research and data.
Strategy Settings
Precursor scoring | Relevance Heuristic |
---|---|
Min. plausibility | 0.01 |
Model | Template_relevance |
Template Set | Pistachio/Bkms_metabolic/Pistachio_ringbreaker/Reaxys/Reaxys_biocatalysis |
Top-N result to add to graph | 6 |
Feasible Synthetic Routes
Disclaimer and Information on In-Vitro Research Products
Please be aware that all articles and product information presented on BenchChem are intended solely for informational purposes. The products available for purchase on BenchChem are specifically designed for in-vitro studies, which are conducted outside of living organisms. In-vitro studies, derived from the Latin term "in glass," involve experiments performed in controlled laboratory settings using cells or tissues. It is important to note that these products are not categorized as medicines or drugs, and they have not received approval from the FDA for the prevention, treatment, or cure of any medical condition, ailment, or disease. We must emphasize that any form of bodily introduction of these products into humans or animals is strictly prohibited by law. It is essential to adhere to these guidelines to ensure compliance with legal and ethical standards in research and experimentation.