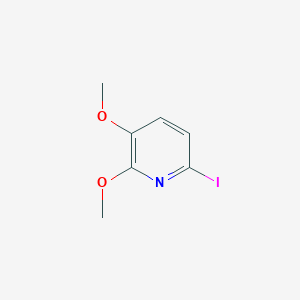
6-Iodo-2,3-dimethoxypyridine
Overview
Description
6-Iodo-2,3-dimethoxypyridine: is a halogenated heterocyclic compound with the molecular formula C7H8INO2 and a molecular weight of 265.05 g/mol . It is characterized by the presence of an iodine atom at the 6th position and two methoxy groups at the 2nd and 3rd positions on the pyridine ring. This compound is of interest in various fields of chemical research due to its unique structural features and reactivity.
Preparation Methods
Synthetic Routes and Reaction Conditions: The synthesis of 6-Iodo-2,3-dimethoxypyridine can be achieved through several methods. One common approach involves the reaction of sodium methoxide with 2-bromo-6-iodo-3-methoxypyridine . The reaction typically proceeds under mild conditions, with the sodium methoxide acting as a base to facilitate the substitution of the bromine atom with a methoxy group.
Industrial Production Methods: While specific industrial production methods for this compound are not widely documented, the general approach would involve scaling up the laboratory synthesis methods. This would include optimizing reaction conditions, such as temperature, pressure, and solvent choice, to ensure high yield and purity of the final product.
Chemical Reactions Analysis
Types of Reactions: 6-Iodo-2,3-dimethoxypyridine undergoes various chemical reactions, including:
Substitution Reactions: The iodine atom can be replaced by other substituents through nucleophilic substitution reactions.
Oxidation and Reduction Reactions: The methoxy groups can participate in oxidation and reduction reactions under appropriate conditions.
Common Reagents and Conditions:
Nucleophilic Substitution: Reagents such as sodium methoxide or other nucleophiles can be used.
Oxidation: Oxidizing agents like potassium permanganate or chromium trioxide.
Reduction: Reducing agents such as lithium aluminum hydride or sodium borohydride.
Major Products: The major products formed from these reactions depend on the specific reagents and conditions used. For example, nucleophilic substitution with sodium methoxide would yield 2,3-dimethoxypyridine derivatives.
Scientific Research Applications
6-Iodo-2,3-dimethoxypyridine has several applications in scientific research:
Chemistry: It is used as a building block in the synthesis of more complex organic molecules.
Biology: It can be used in the study of biological systems and as a potential ligand in biochemical assays.
Medicine: Research into its potential therapeutic applications, such as in the development of new drugs.
Industry: It may be used in the production of specialty chemicals and materials.
Mechanism of Action
The mechanism of action of 6-Iodo-2,3-dimethoxypyridine involves its interaction with various molecular targets. The iodine atom and methoxy groups can participate in hydrogen bonding and other interactions with biological molecules, influencing their activity. The specific pathways and targets depend on the context of its use, such as in biochemical assays or drug development.
Comparison with Similar Compounds
- 5-Iodo-2,3-dimethoxypyridine
- 4-Iodo-2,3-dimethoxypyridine
- 2-Bromo-6-iodo-3,4-dimethoxypyridine
- 2-Iodo-3,5-dimethoxypyridine
Comparison: 6-Iodo-2,3-dimethoxypyridine is unique due to the specific positioning of the iodine atom and methoxy groups, which influence its reactivity and interactions. Compared to its analogs, it may exhibit different chemical and biological properties, making it valuable for specific applications in research and industry .
Properties
IUPAC Name |
6-iodo-2,3-dimethoxypyridine | |
---|---|---|
Source | PubChem | |
URL | https://pubchem.ncbi.nlm.nih.gov | |
Description | Data deposited in or computed by PubChem | |
InChI |
InChI=1S/C7H8INO2/c1-10-5-3-4-6(8)9-7(5)11-2/h3-4H,1-2H3 | |
Source | PubChem | |
URL | https://pubchem.ncbi.nlm.nih.gov | |
Description | Data deposited in or computed by PubChem | |
InChI Key |
FTFRZLORDYKKMJ-UHFFFAOYSA-N | |
Source | PubChem | |
URL | https://pubchem.ncbi.nlm.nih.gov | |
Description | Data deposited in or computed by PubChem | |
Canonical SMILES |
COC1=C(N=C(C=C1)I)OC | |
Source | PubChem | |
URL | https://pubchem.ncbi.nlm.nih.gov | |
Description | Data deposited in or computed by PubChem | |
Molecular Formula |
C7H8INO2 | |
Source | PubChem | |
URL | https://pubchem.ncbi.nlm.nih.gov | |
Description | Data deposited in or computed by PubChem | |
DSSTOX Substance ID |
DTXSID60670127 | |
Record name | 6-Iodo-2,3-dimethoxypyridine | |
Source | EPA DSSTox | |
URL | https://comptox.epa.gov/dashboard/DTXSID60670127 | |
Description | DSSTox provides a high quality public chemistry resource for supporting improved predictive toxicology. | |
Molecular Weight |
265.05 g/mol | |
Source | PubChem | |
URL | https://pubchem.ncbi.nlm.nih.gov | |
Description | Data deposited in or computed by PubChem | |
CAS No. |
321535-23-3 | |
Record name | 6-Iodo-2,3-dimethoxypyridine | |
Source | EPA DSSTox | |
URL | https://comptox.epa.gov/dashboard/DTXSID60670127 | |
Description | DSSTox provides a high quality public chemistry resource for supporting improved predictive toxicology. | |
Synthesis routes and methods I
Procedure details
Synthesis routes and methods II
Procedure details
Synthesis routes and methods III
Procedure details
Retrosynthesis Analysis
AI-Powered Synthesis Planning: Our tool employs the Template_relevance Pistachio, Template_relevance Bkms_metabolic, Template_relevance Pistachio_ringbreaker, Template_relevance Reaxys, Template_relevance Reaxys_biocatalysis model, leveraging a vast database of chemical reactions to predict feasible synthetic routes.
One-Step Synthesis Focus: Specifically designed for one-step synthesis, it provides concise and direct routes for your target compounds, streamlining the synthesis process.
Accurate Predictions: Utilizing the extensive PISTACHIO, BKMS_METABOLIC, PISTACHIO_RINGBREAKER, REAXYS, REAXYS_BIOCATALYSIS database, our tool offers high-accuracy predictions, reflecting the latest in chemical research and data.
Strategy Settings
Precursor scoring | Relevance Heuristic |
---|---|
Min. plausibility | 0.01 |
Model | Template_relevance |
Template Set | Pistachio/Bkms_metabolic/Pistachio_ringbreaker/Reaxys/Reaxys_biocatalysis |
Top-N result to add to graph | 6 |
Feasible Synthetic Routes
Disclaimer and Information on In-Vitro Research Products
Please be aware that all articles and product information presented on BenchChem are intended solely for informational purposes. The products available for purchase on BenchChem are specifically designed for in-vitro studies, which are conducted outside of living organisms. In-vitro studies, derived from the Latin term "in glass," involve experiments performed in controlled laboratory settings using cells or tissues. It is important to note that these products are not categorized as medicines or drugs, and they have not received approval from the FDA for the prevention, treatment, or cure of any medical condition, ailment, or disease. We must emphasize that any form of bodily introduction of these products into humans or animals is strictly prohibited by law. It is essential to adhere to these guidelines to ensure compliance with legal and ethical standards in research and experimentation.