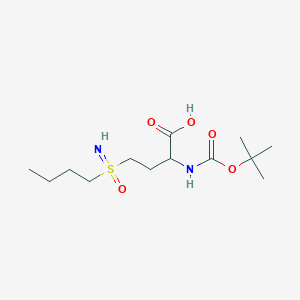
Boc-DL-buthioninesulfoximine
Overview
Description
Boc-DL-buthioninesulfoximine is a synthetic compound widely used in scientific research due to its unique chemical structure and biological activity. It is a potent inhibitor of glutathione synthesis, making it a valuable tool for studying the role of glutathione in various biological processes.
Mechanism of Action
Target of Action
Boc-DL-buthioninesulfoximine primarily targets the enzyme γ-glutamylcysteine synthetase . This enzyme plays a crucial role in the synthesis of glutathione, a vital antioxidant in cells .
Mode of Action
This compound acts by irreversibly inhibiting γ-glutamylcysteine synthetase . This inhibition leads to a decrease in the synthesis of glutathione, thereby inducing oxidative stress within the cell .
Biochemical Pathways
The primary biochemical pathway affected by this compound is the glutathione synthesis pathway . By inhibiting γ-glutamylcysteine synthetase, this compound disrupts the production of glutathione. This disruption can lead to an increase in reactive oxygen species within the cell, potentially leading to oxidative damage .
Pharmacokinetics
The compound’s molecular weight (32243) and predicted density (121 g/cm^3) may influence its bioavailability .
Result of Action
The primary result of this compound’s action is the induction of oxidative stress within the cell due to glutathione depletion . This oxidative stress can lead to DNA deletions, which may play a role in carcinogenesis .
Action Environment
The action of this compound can be influenced by various environmental factors. For instance, the pH of the environment could potentially affect the stability and efficacy of the compound . .
Biochemical Analysis
Biochemical Properties
Boc-DL-buthioninesulfoximine plays a crucial role in biochemical reactions by inhibiting the enzyme γ-glutamylcysteine synthetase, which is essential for the synthesis of glutathione . Glutathione is a vital antioxidant that protects cells from oxidative damage. By inhibiting its synthesis, this compound helps researchers study the effects of reduced glutathione levels on various cellular processes. The compound interacts with γ-glutamylcysteine synthetase by binding to its active site, thereby preventing the enzyme from catalyzing the formation of γ-glutamylcysteine, a precursor of glutathione .
Cellular Effects
This compound has significant effects on various types of cells and cellular processes. By depleting glutathione levels, it induces oxidative stress, which can lead to cell death or apoptosis in certain cell types . This compound has been shown to enhance the antitumor effects of other treatments, such as ionizing radiation and chemotherapeutic agents, by increasing the sensitivity of cancer cells to oxidative damage . Additionally, this compound affects cell signaling pathways, gene expression, and cellular metabolism by altering the redox state of the cell .
Molecular Mechanism
The molecular mechanism of this compound involves its interaction with γ-glutamylcysteine synthetase, leading to the inhibition of glutathione synthesis . This inhibition results in a decrease in intracellular glutathione levels, which in turn increases the levels of reactive oxygen species (ROS) within the cell . The elevated ROS levels cause oxidative damage to cellular components, including DNA, proteins, and lipids, ultimately leading to cell death or apoptosis . This compound also affects gene expression by modulating the activity of redox-sensitive transcription factors .
Temporal Effects in Laboratory Settings
In laboratory settings, the effects of this compound can change over time. The compound is relatively stable under standard storage conditions, but its activity may decrease over prolonged periods . In in vitro studies, Boc
Preparation Methods
Synthetic Routes and Reaction Conditions
The synthesis of Boc-DL-buthioninesulfoximine typically involves the protection of the amino group of buthionine sulfoximine with a tert-butyloxycarbonyl (Boc) group. This protection is achieved through a reaction with di-tert-butyl dicarbonate in the presence of a base such as triethylamine. The reaction is carried out under anhydrous conditions to prevent hydrolysis of the Boc group .
Industrial Production Methods
the synthesis generally follows similar principles as laboratory-scale synthesis, with optimization for larger-scale production, including the use of more efficient reaction conditions and purification techniques .
Chemical Reactions Analysis
Types of Reactions
Boc-DL-buthioninesulfoximine undergoes various chemical reactions, including:
Oxidation: The sulfoximine group can be oxidized to form sulfone derivatives.
Reduction: The sulfoximine group can be reduced to form sulfide derivatives.
Substitution: The Boc group can be removed under acidic conditions to yield the free amine.
Common Reagents and Conditions
Oxidation: Common oxidizing agents include hydrogen peroxide and m-chloroperbenzoic acid.
Reduction: Common reducing agents include lithium aluminum hydride and sodium borohydride.
Substitution: Acidic conditions, such as treatment with trifluoroacetic acid, are used to remove the Boc group.
Major Products Formed
Oxidation: Sulfone derivatives.
Reduction: Sulfide derivatives.
Substitution: Free amine.
Scientific Research Applications
Boc-DL-buthioninesulfoximine has a wide range of applications in scientific research, including:
Chemistry: Used as a reagent in the synthesis of other compounds.
Biology: Used to study the role of glutathione in cellular processes.
Medicine: Investigated for its potential in cancer therapy due to its ability to deplete glutathione levels in cancer cells.
Industry: Used in the development of new drugs and therapeutic agents
Comparison with Similar Compounds
Similar Compounds
L-buthionine sulfoximine: Similar in structure but lacks the Boc protection group.
DL-buthionine sulfoximine: Similar in structure but lacks the Boc protection group.
S-buthionine sulfoximine: Similar in structure but has a different stereochemistry.
Uniqueness
Boc-DL-buthioninesulfoximine is unique due to the presence of the Boc protection group, which enhances its stability and allows for selective deprotection under acidic conditions. This makes it a versatile reagent in synthetic chemistry and a valuable tool in biological research .
Properties
IUPAC Name |
4-(butylsulfonimidoyl)-2-[(2-methylpropan-2-yl)oxycarbonylamino]butanoic acid | |
---|---|---|
Source | PubChem | |
URL | https://pubchem.ncbi.nlm.nih.gov | |
Description | Data deposited in or computed by PubChem | |
InChI |
InChI=1S/C13H26N2O5S/c1-5-6-8-21(14,19)9-7-10(11(16)17)15-12(18)20-13(2,3)4/h10,14H,5-9H2,1-4H3,(H,15,18)(H,16,17) | |
Source | PubChem | |
URL | https://pubchem.ncbi.nlm.nih.gov | |
Description | Data deposited in or computed by PubChem | |
InChI Key |
IIJKAKPMCGGPSE-UHFFFAOYSA-N | |
Source | PubChem | |
URL | https://pubchem.ncbi.nlm.nih.gov | |
Description | Data deposited in or computed by PubChem | |
Canonical SMILES |
CCCCS(=N)(=O)CCC(C(=O)O)NC(=O)OC(C)(C)C | |
Source | PubChem | |
URL | https://pubchem.ncbi.nlm.nih.gov | |
Description | Data deposited in or computed by PubChem | |
Molecular Formula |
C13H26N2O5S | |
Source | PubChem | |
URL | https://pubchem.ncbi.nlm.nih.gov | |
Description | Data deposited in or computed by PubChem | |
Molecular Weight |
322.42 g/mol | |
Source | PubChem | |
URL | https://pubchem.ncbi.nlm.nih.gov | |
Description | Data deposited in or computed by PubChem | |
Retrosynthesis Analysis
AI-Powered Synthesis Planning: Our tool employs the Template_relevance Pistachio, Template_relevance Bkms_metabolic, Template_relevance Pistachio_ringbreaker, Template_relevance Reaxys, Template_relevance Reaxys_biocatalysis model, leveraging a vast database of chemical reactions to predict feasible synthetic routes.
One-Step Synthesis Focus: Specifically designed for one-step synthesis, it provides concise and direct routes for your target compounds, streamlining the synthesis process.
Accurate Predictions: Utilizing the extensive PISTACHIO, BKMS_METABOLIC, PISTACHIO_RINGBREAKER, REAXYS, REAXYS_BIOCATALYSIS database, our tool offers high-accuracy predictions, reflecting the latest in chemical research and data.
Strategy Settings
Precursor scoring | Relevance Heuristic |
---|---|
Min. plausibility | 0.01 |
Model | Template_relevance |
Template Set | Pistachio/Bkms_metabolic/Pistachio_ringbreaker/Reaxys/Reaxys_biocatalysis |
Top-N result to add to graph | 6 |
Feasible Synthetic Routes
Disclaimer and Information on In-Vitro Research Products
Please be aware that all articles and product information presented on BenchChem are intended solely for informational purposes. The products available for purchase on BenchChem are specifically designed for in-vitro studies, which are conducted outside of living organisms. In-vitro studies, derived from the Latin term "in glass," involve experiments performed in controlled laboratory settings using cells or tissues. It is important to note that these products are not categorized as medicines or drugs, and they have not received approval from the FDA for the prevention, treatment, or cure of any medical condition, ailment, or disease. We must emphasize that any form of bodily introduction of these products into humans or animals is strictly prohibited by law. It is essential to adhere to these guidelines to ensure compliance with legal and ethical standards in research and experimentation.