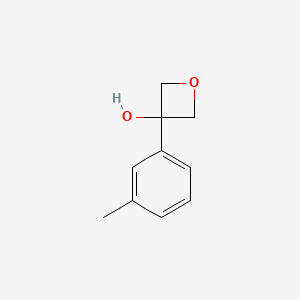
3-(M-Tolyl)oxetan-3-OL
- Click on QUICK INQUIRY to receive a quote from our team of experts.
- With the quality product at a COMPETITIVE price, you can focus more on your research.
Overview
Description
3-(M-Tolyl)oxetan-3-OL is a cyclic ether alcohol featuring an oxetane ring substituted with a meta-tolyl group. Oxetanes, particularly oxetan-3-ol derivatives, are of significant interest in medicinal chemistry due to their role as bioisosteres for carboxylic acids and other polar functional groups. The oxetane ring introduces rigidity and modulates physicochemical properties such as solubility, metabolic stability, and hydrogen-bonding capacity, making it a valuable scaffold in drug design .
Preparation Methods
Synthetic Routes and Reaction Conditions
The synthesis of 3-(M-Tolyl)oxetan-3-OL typically involves the formation of the oxetane ring through cyclization reactions. One common method is the intramolecular cyclization of epoxides. For instance, the reaction of 2-bromobenzoic acid with terminal epoxides in the presence of tetrabutylammonium bromide as a catalyst can yield oxetan-3-ol derivatives . Another approach involves the use of epichlorohydrin as a starting material, which undergoes ring-opening and subsequent cyclization to form the oxetane ring .
Industrial Production Methods
Industrial production of this compound may involve optimized versions of the synthetic routes mentioned above. The use of phase-transfer catalysts and controlled reaction conditions can enhance the yield and selectivity of the desired product. The scalability of these methods allows for the efficient production of oxetane derivatives on an industrial scale.
Chemical Reactions Analysis
Types of Reactions
3-(M-Tolyl)oxetan-3-OL undergoes various chemical reactions, including:
Oxidation: The oxetane ring can be oxidized to form corresponding oxetanones or other oxidized derivatives.
Reduction: Reduction reactions can convert oxetane derivatives into alcohols or other reduced forms.
Substitution: Nucleophilic substitution reactions can occur at the oxetane ring, leading to the formation of substituted oxetanes.
Common Reagents and Conditions
Oxidation: Common oxidizing agents include peroxy acids and hydrogen peroxide.
Reduction: Reducing agents such as lithium aluminum hydride (LiAlH4) or sodium borohydride (NaBH4) are often used.
Substitution: Nucleophiles like amines, thiols, and halides can be employed under appropriate conditions.
Major Products Formed
The major products formed from these reactions depend on the specific reagents and conditions used. For example, oxidation of this compound can yield oxetanones, while reduction can produce alcohols.
Scientific Research Applications
3-(M-Tolyl)oxetan-3-OL has a wide range of applications in scientific research:
Mechanism of Action
The mechanism of action of 3-(M-Tolyl)oxetan-3-OL involves its interaction with molecular targets and pathways. The strained oxetane ring can undergo ring-opening reactions, leading to the formation of reactive intermediates that can interact with various biological targets. These interactions can modulate enzymatic activities, receptor binding, and other cellular processes .
Comparison with Similar Compounds
Comparison with Structurally Similar Compounds
Oxetane-Based Derivatives
Oxetan-3-ol (C₃H₆O₂, CAS 7748-36-9)
- Structure : Simplest oxetane alcohol.
- Physicochemical Properties : Lower molecular weight (74.08 g/mol) and higher polarity compared to 3-(M-Tolyl)oxetan-3-OL.
- Research Findings : Demonstrated efficacy in ibuprofen derivatives, retaining cyclooxygenase inhibition despite lacking a carboxylic acid group .
3-Ethynyloxetan-3-ol (C₅H₆O₂)
- Structure : Ethynyl substitution enhances reactivity for click chemistry applications.
- Key Data : Predicted collision cross-section (CCS) values for adducts (e.g., [M+H]+: 115.3 Ų) suggest a compact, rigid structure, contrasting with the bulkier m-tolyl variant .
This compound vs. Thietan-3-ol (C₃H₆OS)
- Structural Difference : Thietan-3-ol replaces the oxygen atom in oxetane with sulfur.
- Studies indicate thietan-3-ol derivatives exhibit reduced metabolic stability compared to oxetane analogs .
Non-Cyclic Alcohols
1-Octen-3-ol (C₈H₁₆O, CAS 3391-86-4)
- Structure : Linear unsaturated alcohol.
- Safety Profile : Requires stringent ventilation and fire hazard precautions, unlike oxetanes, which are less volatile due to ring strain .
3-Methyl-3-octanol (C₉H₂₀O, CAS 5340-36-3)
- Structure : Branched aliphatic alcohol.
- Applications : Used in fragrances and solvents. Higher logP (3.1 estimated) compared to this compound, suggesting poorer aqueous solubility .
Functional Group Bioisosteres
Carboxylic Acids (e.g., Ibuprofen)
- Comparison : this compound eliminates ionization (pKa ~4.7 for carboxylic acids vs. ~14 for alcohols), improving membrane permeability. In vitro studies show oxetane-based ibuprofen analogs retain ~70% COX-2 inhibition efficacy .
Tetrazoles and Sulfonamides
- Key Differences : Tetrazoles (pKa ~5) mimic carboxylic acid ionization but introduce nitrogen-rich moieties, which may affect toxicity. Sulfonamides offer hydrogen-bonding but lack the conformational constraint of oxetanes .
Data Tables
Table 1: Physicochemical Comparison of Selected Compounds
Compound | Molecular Formula | Molecular Weight (g/mol) | logP* | Key Feature |
---|---|---|---|---|
This compound | C₁₀H₁₂O₂ | 164.20 | 1.8 | Rigid, bioisostere |
Oxetan-3-ol | C₃H₆O₂ | 74.08 | -0.5 | High polarity |
Thietan-3-ol | C₃H₆OS | 90.14 | 0.2 | Sulfur substitution |
Ibuprofen | C₁₃H₁₈O₂ | 206.28 | 3.5 | Carboxylic acid comparator |
*Estimated using fragment-based methods.
Research Implications
- Drug Design : this compound offers a balance between polarity and lipophilicity, enhancing bioavailability compared to carboxylic acids.
- Metabolism : Oxetanes generally exhibit slower hepatic clearance than thietanes or linear alcohols due to reduced enzymatic recognition .
Biological Activity
3-(M-Tolyl)oxetan-3-OL is an organic compound characterized by its oxetane ring, which is a four-membered cyclic ether. The presence of a methyl-substituted phenyl ring significantly influences its chemical reactivity and biological activity. This article discusses the biological activity of this compound, its mechanisms of action, and relevant research findings.
Property | Value |
---|---|
Molecular Formula | C10H12O2 |
Molecular Weight | 164.20 g/mol |
IUPAC Name | 3-(3-methylphenyl)oxetan-3-ol |
InChI Key | LZIXJNQKAVPEGF-UHFFFAOYSA-N |
The unique structure of this compound allows it to participate in various chemical reactions, including oxidation, reduction, and nucleophilic substitution, making it a valuable intermediate in organic synthesis and drug development.
The biological activity of this compound is attributed to its interaction with molecular targets within biological systems. The strained oxetane ring can undergo ring-opening reactions, producing reactive intermediates that may interact with enzymes and receptors, thereby modulating various cellular processes .
Inhibition of Eicosanoid Biosynthesis
Research has shown that oxetane derivatives, including this compound, can serve as bioisosteres for carboxylic acids. A study evaluating the inhibition of cyclooxygenase (COX) and lipoxygenase (5-LOX) pathways revealed that these compounds could inhibit the production of eicosanoids in RBL-1 cells. The IC50 values for various derivatives indicated significant inhibition potential, suggesting that this compound may be effective in modulating inflammatory responses .
Cytotoxicity Against Cancer Cell Lines
Recent studies have also assessed the cytotoxic effects of oxetane-containing compounds against human cancer cell lines. For instance, new analogues derived from oxetanes demonstrated potent cytotoxicity against MCF-7 and other cancer cell lines with IC50 values as low as 0.47 μM . This suggests potential applications in cancer therapeutics.
Case Studies
- Inhibition Studies : In a study involving RBL-1 cells, the compound was tested for its ability to inhibit COX-derived prostaglandins (PGD2 and PGE2) and 5-LOX-derived leukotrienes (LTB4). The results highlighted that certain oxetane derivatives exhibited balanced inhibition across both pathways, indicating their potential as anti-inflammatory agents .
- Cancer Research : Another study focused on synthesizing oxetane analogues for evaluating their cytotoxic effects against breast cancer cell lines. The findings revealed that while some compounds did not effectively inhibit tubulin polymerization, they displayed significant cytotoxicity, suggesting alternative mechanisms of action through which these compounds may exert their effects .
Q & A
Basic Research Questions
Q. What are the common synthetic methodologies for preparing 3-(M-Tolyl)oxetan-3-OL, and what experimental precautions are critical?
- Answer : The synthesis often involves Friedel-Crafts alkylation or lithiation-mediated coupling. For example, lithiation of a substituted aryl bromide (e.g., 5-bromo-1,3-benzodioxole) with n-BuLi in THF at low temperatures (–70°C), followed by reaction with oxetan-3-one derivatives . Precautions include strict temperature control, inert atmosphere (argon/nitrogen), and handling pyrophoric reagents (e.g., n-BuLi) in flame-dried glassware. Safety protocols for flammable solvents (THF) and corrosive reagents (SOCl₂) must align with OSHA guidelines, including PPE (gloves, goggles) and fume hood use .
Q. How can researchers verify the structural integrity of this compound post-synthesis?
- Answer : Use a combination of spectroscopic techniques:
- NMR : Compare δ values for oxetane protons (typically 4.5–5.0 ppm) and aryl groups with literature data .
- HRMS : Confirm molecular ion peaks (e.g., m/z 248.05 for a related bromo-fluoro analog) .
- XRD : Resolve stereochemistry if crystallized. Cross-reference with databases (e.g., Cambridge Structural Database) to validate geometry .
Q. What storage and handling protocols are recommended for this compound?
- Answer : Store in airtight, light-resistant containers under inert gas (argon) at –20°C to prevent oxidation. Avoid proximity to acids/bases, as oxetanes may undergo ring-opening. For handling, use nitrile gloves and conduct experiments in ventilated areas to minimize inhalation risks .
Q. How does the presence of substituents (e.g., methyl, halogens) on the aryl ring affect the compound’s reactivity?
- Answer : Electron-donating groups (e.g., methyl) enhance Friedel-Crafts alkylation yields by activating the aryl ring, while electron-withdrawing groups (e.g., halogens) may require harsher conditions (e.g., higher temps or Lewis acids like ZnCl₂). Steric effects from ortho-substituents can reduce reaction efficiency .
Q. What analytical techniques are suitable for assessing purity, and what thresholds are acceptable for biological assays?
- Answer : HPLC (≥95% purity, using C18 columns and UV detection at 254 nm) is standard. Impurity profiling via LC-MS identifies byproducts (e.g., ring-opened derivatives). For in vitro assays, purity ≥95% minimizes off-target effects .
Advanced Research Questions
Q. How can this compound serve as a bioisostere for carboxylic acids, and what experimental strategies validate this application?
- Answer : The oxetane’s polarity and hydrogen-bonding capacity mimic carboxylic acids. To evaluate bioisosterism:
- Computational modeling : Compare electrostatic potential maps and pKa values (oxetane ~14 vs. carboxylic acid ~4–5) .
- In vitro assays : Test binding affinity in enzyme inhibition studies (e.g., kinase assays) and measure solubility/logP to assess pharmacokinetic improvements .
Q. What factors influence regioselectivity in Friedel-Crafts reactions involving this compound derivatives?
- Answer : Regioselectivity depends on:
- Catalyst : LiCl/ZnCl₂ mixtures favor diarylation at the oxetane’s 3-position .
- Solvent polarity : Polar aprotic solvents (THF) stabilize transition states for para-substitution.
- Directing groups : Pyridinyl or benzodioxolyl substituents guide electrophilic attack via coordination to Lewis acids .
Q. How should researchers address contradictory data in reaction yields or spectroscopic results across studies?
- Answer : Systematically analyze variables:
- Reaction conditions : Trace moisture or oxygen can deactivate catalysts (e.g., n-BuLi), leading to low yields. Replicate studies under rigorously anhydrous conditions .
- Analytical methods : Ensure NMR spectra are referenced to internal standards (e.g., TMS) and acquired at consistent magnetic field strengths. Cross-validate with independent techniques (e.g., IR for functional groups) .
Q. What strategies optimize the metabolic stability of this compound in preclinical ADME studies?
- Answer :
- Microsomal stability assays : Incubate with liver microsomes (human/rat) to identify metabolic hotspots (e.g., oxetane ring oxidation). Introduce deuterium at labile positions to slow degradation .
- Caco-2 permeability : Measure apparent permeability (Papp) to assess oral bioavailability. Modify logP via fluorination or methyl group addition to enhance membrane penetration .
Q. How can computational tools predict the synthetic accessibility of novel this compound analogs?
Properties
Molecular Formula |
C10H12O2 |
---|---|
Molecular Weight |
164.20 g/mol |
IUPAC Name |
3-(3-methylphenyl)oxetan-3-ol |
InChI |
InChI=1S/C10H12O2/c1-8-3-2-4-9(5-8)10(11)6-12-7-10/h2-5,11H,6-7H2,1H3 |
InChI Key |
LZIXJNQKAVPEGF-UHFFFAOYSA-N |
Canonical SMILES |
CC1=CC(=CC=C1)C2(COC2)O |
Origin of Product |
United States |
Disclaimer and Information on In-Vitro Research Products
Please be aware that all articles and product information presented on BenchChem are intended solely for informational purposes. The products available for purchase on BenchChem are specifically designed for in-vitro studies, which are conducted outside of living organisms. In-vitro studies, derived from the Latin term "in glass," involve experiments performed in controlled laboratory settings using cells or tissues. It is important to note that these products are not categorized as medicines or drugs, and they have not received approval from the FDA for the prevention, treatment, or cure of any medical condition, ailment, or disease. We must emphasize that any form of bodily introduction of these products into humans or animals is strictly prohibited by law. It is essential to adhere to these guidelines to ensure compliance with legal and ethical standards in research and experimentation.