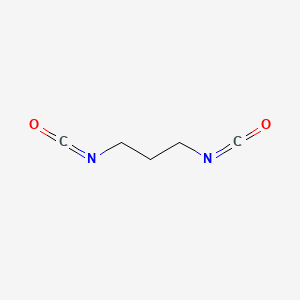
Propane, 1,3-diisocyanato-
- Click on QUICK INQUIRY to receive a quote from our team of experts.
- With the quality product at a COMPETITIVE price, you can focus more on your research.
Overview
Description
1,3-Diisocyanatopropane is an organic compound with the molecular formula C5H6N2O2. It is a member of the isocyanate family, characterized by the presence of two isocyanate groups (-N=C=O) attached to a propane backbone. This compound is known for its reactivity and is used in various industrial and research applications .
Preparation Methods
1,3-Diisocyanatopropane can be synthesized through several methods. One common synthetic route involves the reaction of 1,3-diaminopropane with phosgene. The reaction typically occurs under controlled conditions to ensure the safe handling of phosgene, a toxic and hazardous reagent . The general reaction is as follows:
H2N−(CH2)3−NH2+2COCl2→O=C=N−(CH2)3−N=C=O+4HCl
Industrial production methods often involve similar reactions but on a larger scale, with additional steps for purification and stabilization of the final product .
Chemical Reactions Analysis
1,3-Diisocyanatopropane undergoes various chemical reactions, primarily due to the reactivity of its isocyanate groups. Some of the key reactions include:
Addition Reactions: Isocyanates readily react with alcohols to form urethanes and with amines to form ureas.
Hydrolysis: In the presence of water, isocyanates hydrolyze to form amines and carbon dioxide.
Polymerization: 1,3-Diisocyanatopropane can polymerize with diols or diamines to form polyurethanes or polyureas, respectively.
Scientific Research Applications
1,3-Diisocyanatopropane is utilized in various scientific research fields:
Mechanism of Action
The reactivity of 1,3-diisocyanatopropane is primarily due to its isocyanate groups. These groups can form covalent bonds with nucleophiles such as alcohols, amines, and water. The mechanism involves the nucleophilic attack on the carbon atom of the isocyanate group, leading to the formation of urethanes, ureas, or amines . This reactivity is exploited in various applications, from polymer synthesis to biological studies.
Comparison with Similar Compounds
1,3-Diisocyanatopropane can be compared with other isocyanates such as:
1,6-Diisocyanatohexane: Used in the production of polyurethanes, it has a longer carbon chain, which affects the properties of the resulting polymers.
Toluene Diisocyanate: Commonly used in flexible foam production, it has aromatic rings that influence its reactivity and the properties of the polymers formed.
Methylenediphenyl Diisocyanate: Used in rigid foam production, it has a more complex structure that provides different mechanical properties to the polymers.
1,3-Diisocyanatopropane is unique due to its shorter carbon chain and the presence of two isocyanate groups, making it highly reactive and suitable for specific applications in polymer chemistry and material science .
Biological Activity
Propane, 1,3-diisocyanato- (also known as 1,3-diphenylurea) is a diisocyanate compound with significant industrial applications, particularly in the production of polyurethane materials. However, its biological activity is primarily characterized by its potential toxicity and allergenic properties. This article provides a detailed overview of its biological activity, including toxicity data, case studies, and research findings.
- Chemical Formula : C5H6N2O2
- Molecular Weight : 126.11 g/mol
- Functional Groups : Contains two isocyanate (-NCO) groups.
The presence of two isocyanate groups enhances the compound's reactivity and ability to form cross-linked structures, making it valuable in various industrial applications such as foams, elastomers, and coatings.
Toxicity and Health Risks
The biological activity of propane, 1,3-diisocyanato- is primarily linked to its toxicity. Key health risks associated with exposure include:
- Respiratory Issues : Inhalation of diisocyanates can lead to respiratory sensitization and asthma. Studies have shown that exposure can provoke allergic reactions in susceptible individuals .
- Skin Sensitization : Contact with skin can lead to dermatitis and other allergic reactions due to the compound's ability to bind covalently with proteins .
- Central Nervous System Effects : High levels of exposure may result in CNS depression .
Exposure Routes
The primary routes of exposure include:
- Inhalation : Common in occupational settings where diisocyanates are used.
- Dermal Contact : Occurs during handling or spills.
- Ingestion : Less common but possible through contaminated food or water.
Respiratory Sensitization
A notable case involved workers in a polyurethane manufacturing facility who developed occupational asthma after prolonged exposure to diisocyanates. The study highlighted the importance of monitoring air quality and implementing safety measures to minimize inhalation risks .
Skin Reactions
Another case study documented a worker who experienced severe dermatitis after handling products containing propane, 1,3-diisocyanato-. Patch testing confirmed sensitization to the compound, underscoring the need for protective gear when working with diisocyanates.
Mechanisms of Toxicity
Research indicates that the mechanism of toxicity involves:
- Covalent Bonding : Diisocyanates react with nucleophilic sites on proteins and other biomolecules, leading to the formation of hapten-protein complexes that trigger immune responses .
- Inflammatory Responses : Exposure can cause inflammation in respiratory tissues, contributing to chronic respiratory conditions .
Comparative Toxicity Studies
A comparative analysis of various diisocyanates revealed that propane, 1,3-diisocyanato- exhibited similar allergenic properties to other common diisocyanates like toluene diisocyanate (TDI) and methylene diphenyl diisocyanate (MDI). However, the specific reactivity and sensitization potential can vary significantly among these compounds .
Data Table: Toxicological Effects Summary
Properties
CAS No. |
3753-93-3 |
---|---|
Molecular Formula |
C5H6N2O2 |
Molecular Weight |
126.11 g/mol |
IUPAC Name |
1,3-diisocyanatopropane |
InChI |
InChI=1S/C5H6N2O2/c8-4-6-2-1-3-7-5-9/h1-3H2 |
InChI Key |
IKYNWXNXXHWHLL-UHFFFAOYSA-N |
Canonical SMILES |
C(CN=C=O)CN=C=O |
Origin of Product |
United States |
Disclaimer and Information on In-Vitro Research Products
Please be aware that all articles and product information presented on BenchChem are intended solely for informational purposes. The products available for purchase on BenchChem are specifically designed for in-vitro studies, which are conducted outside of living organisms. In-vitro studies, derived from the Latin term "in glass," involve experiments performed in controlled laboratory settings using cells or tissues. It is important to note that these products are not categorized as medicines or drugs, and they have not received approval from the FDA for the prevention, treatment, or cure of any medical condition, ailment, or disease. We must emphasize that any form of bodily introduction of these products into humans or animals is strictly prohibited by law. It is essential to adhere to these guidelines to ensure compliance with legal and ethical standards in research and experimentation.