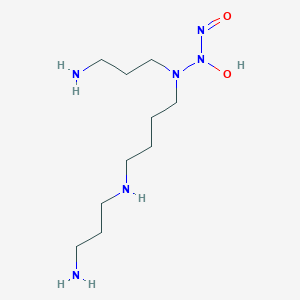
Spermine NONOate
Overview
Description
Spermine NONOate is a diazeniumdiolate compound that serves as a nitric oxide donor. Nitric oxide is a simple and unstable free radical molecule that plays a crucial role in various physiological processes, including vasodilation, neurotransmission, and immune response . This compound releases nitric oxide spontaneously under physiological conditions, making it a valuable tool in scientific research and medical applications .
Preparation Methods
Spermine NONOate can be synthesized by reacting spermine with nitric oxide under specific conditions. The reaction typically involves the use of a solvent such as methanol or ethanol and is carried out at low temperatures to ensure the stability of the product . The resulting compound is a white solid that is soluble in water and other polar solvents .
Chemical Reactions Analysis
Spermine NONOate undergoes spontaneous dissociation to release nitric oxide and the corresponding amine. This dissociation is pH-dependent and occurs more rapidly under acidic conditions . The primary reaction involves the cleavage of the diazeniumdiolate group, resulting in the formation of two molecules of nitric oxide and one molecule of spermine . This reaction is commonly used in biological studies to generate controlled amounts of nitric oxide.
Scientific Research Applications
Biochemical Research
Protein Dynamics and Stability
Spermine NONOate has been extensively studied for its effects on protein stability and dynamics. Research indicates that it can significantly alter the unfolding temperature () of proteins such as hemoglobin. For example, a study demonstrated that the addition of this compound decreased the unfolding temperature of hemoglobin in both sodium and potassium buffers, suggesting that it influences the thermal stability of proteins through NO-mediated pathways .
Table 1: Effects of this compound on Hemoglobin Unfolding Temperature
Buffer Type | Control (°C) | with this compound (°C) | Effect |
---|---|---|---|
Sodium | 64.0 ± 0.6 | 56.0 ± 1.3 | Decrease |
Potassium | 62.0 ± 1.1 | 59.0 ± 1.7 | Decrease |
Mechanism of Action
The mechanism by which this compound exerts its effects involves the generation of reactive nitrogen species, which can modify amino acid residues in proteins, particularly tryptophan . This modification can lead to changes in protein conformation and function.
Pharmacological Applications
Therapeutic Potential
This compound has shown promise in various therapeutic applications, including cardiovascular health and erectile dysfunction treatment. Its ability to release NO can lead to vasodilation, improving blood flow and potentially alleviating conditions related to impaired nitric oxide signaling .
Case Study: Erectile Dysfunction
Research has indicated that this compound may be beneficial in treating erectile dysfunction, as it enhances NO availability, which is crucial for the physiological processes involved in achieving an erection . This application highlights its potential role in pharmacotherapy.
Cellular Signaling
Role in Neurotransmission
This compound is also used in studies examining its role in neurotransmission and neuroprotection. The compound's ability to modulate neuronal signaling pathways through NO release makes it a valuable tool for understanding neurobiological processes .
Case Study: Neuroprotective Effects
In experimental models, this compound has been shown to exert neuroprotective effects by enhancing synaptic plasticity and reducing oxidative stress within neuronal cells . These findings suggest its potential application in neurodegenerative disease research.
Nitric Oxide Measurement
Quantification Techniques
This compound is frequently utilized in studies involving the measurement of NO levels within biological samples. The Griess reagent assay is commonly employed to quantify nitrite levels as an indirect measure of NO production following the decomposition of this compound .
Table 2: Nitric Oxide Measurement Techniques
Technique | Description |
---|---|
Griess Reagent Assay | Measures nitrite levels as an indicator of NO release |
Fluorescent Probes | Detects reactive nitrogen species in live cells |
Mechanism of Action
The mechanism of action of Spermine NONOate involves the spontaneous release of nitric oxide, which then diffuses into surrounding tissues and cells. Nitric oxide interacts with various molecular targets, including guanylate cyclase, leading to the production of cyclic guanosine monophosphate (cGMP). This signaling molecule mediates various physiological effects, such as vasodilation and inhibition of platelet aggregation . Nitric oxide also acts as a free radical scavenger, protecting cells from oxidative damage .
Comparison with Similar Compounds
Similar compounds include diethylamine NONOate, proline NONOate, and methylamine NONOate . Each of these compounds has unique properties in terms of nitric oxide release rates and stability. For example, diethylamine NONOate releases nitric oxide more rapidly than Spermine NONOate, making it suitable for applications requiring quick nitric oxide delivery . In contrast, this compound provides a more sustained release, making it ideal for studies requiring prolonged nitric oxide exposure .
Biological Activity
Spermine NONOate (SNN) is a nitric oxide (NO) donor that has garnered attention for its diverse biological activities, particularly its role in modulating oxidative stress, affecting protein stability, and exhibiting anticoagulant properties. This article synthesizes findings from various studies to provide a comprehensive overview of the biological activity of this compound.
This compound is a stable complex of spermine and nitric oxide, designed for controlled release of NO. Its chemical structure is represented as:
The compound has a half-life of approximately 39 minutes at 37°C and pH 7.4, with an effective concentration (EC50) of 6.2 μM for inducing relaxation in rabbit aorta tissues . The release of NO from SNN plays a crucial role in its biological effects, including vasodilation and modulation of cellular signaling pathways.
Antioxidant Activity
This compound has demonstrated significant antioxidant properties, particularly in the context of low-density lipoprotein (LDL) oxidation. Research indicates that SNN inhibits copper(II) sulfate-induced oxidative modification of LDL by reducing the formation of thiobarbituric acid reactive substances and conjugated diene formation . The inhibitory effect is concentration-dependent, with low concentrations being less effective compared to higher doses (above 4 μM), which show a marked enhancement in antioxidant activity .
Effects on Protein Stability
A study investigated the impact of this compound on the thermal stability of human hemoglobin. The findings revealed that SNN significantly decreased the unfolding temperature () of hemoglobin, suggesting that NO can destabilize protein structures under certain conditions. For example, in sodium-based buffers, the was reduced from 64.0°C to 56.0°C upon addition of SNN . This effect was influenced by the ionic composition of the buffer, highlighting the complex interactions between NO donors and protein stability.
Table 1: Effects of this compound on Hemoglobin Unfolding Temperature
Buffer Type | Control (°C) | with SNN (°C) | Change (°C) |
---|---|---|---|
Sodium | 64.0 ± 0.6 | 56.0 ± 1.3 | -8.0 |
Potassium | 62.0 ± 1.1 | 59.0 ± 1.7 | -3.0 |
Anticoagulant Activity
This compound also exhibits anticoagulant properties. Studies have shown that it can enhance the anticoagulant effects when used in conjunction with heparin, demonstrating potential for therapeutic applications in managing coagulation disorders . The half-life at physiological conditions allows for sustained anticoagulant activity, making it a candidate for further investigation in clinical settings.
Case Studies and Clinical Implications
Case Study: Neuroprotection
In a study examining neuroprotection against oxidative stress in neuronal cells, SNN was shown to promote cell survival under conditions mimicking neurodegenerative diseases . The study indicated that SNN could mitigate cognitive dysfunction associated with Alzheimer's disease by reducing oxidative damage and enhancing neuronal health.
Case Study: Bacterial Resistance
Another investigation revealed that SNN could protect bacteria from aminoglycoside antibiotics by inhibiting respiration in Salmonella species . This finding suggests a dual role for NO donors in both promoting and inhibiting microbial activity depending on the context.
Q & A
Basic Research Questions
Q. What are the primary mechanisms of action of Spermine NONOate in vascular relaxation, and how should experimental conditions be optimized to study these pathways?
this compound acts as a nitric oxide (NO) donor, inducing vascular relaxation through both cGMP-dependent and -independent pathways. In rat pulmonary arteries, its cGMP-independent effects involve activation of Na+/K+-ATPase, sarcoplasmic reticulum Ca²⁺-ATPase (SERCA), and calcium-activated potassium channels (KCa) . To study these pathways:
- Experimental Design : Use inhibitors like ODQ (10 μM) to block soluble guanylate cyclase (sGC) and isolate cGMP-independent effects. Pre-contract tissues with phenylephrine (0.1 μM) to assess relaxation responses .
- Controls : Include potassium channel blockers (e.g., charybdotoxin, 300 nM) or ouabain (1 mM) to validate pathway-specific contributions .
- Buffer Conditions : Maintain pH 7.0–7.4 and temperature at 37°C, as NO release kinetics are pH- and temperature-dependent .
Q. What factors influence the stability and NO release kinetics of this compound, and how can researchers control these variables in physiological studies?
- pH Sensitivity : this compound decomposes rapidly at acidic pH (half-life <1 min at pH 5.1) but has a half-life of 39 min at pH 7.4 (37°C). Use phosphate-buffered saline (PBS, pH 7.4) for consistent NO release .
- Temperature : Store aliquots at -80°C for long-term stability; avoid repeated freeze-thaw cycles. Prepare fresh working solutions for each experiment .
- Measurement Tools : Quantify NO release indirectly via Griess assay (measuring nitrite levels) or directly using amperometric NO sensors .
Advanced Research Questions
Q. How can researchers resolve contradictions between observed cGMP-independent relaxation and measured cGMP elevations caused by this compound in vascular models?
Despite this compound increasing cGMP levels 35-fold in rat pulmonary arteries, ODQ (3 μM) blocks cGMP production but not relaxation, indicating cGMP-independent mechanisms . To address this contradiction:
- Methodological Approach :
- Data Interpretation : Analyze relaxation curves and cGMP levels in parallel. A lack of correlation suggests alternative pathways (e.g., direct activation of ion pumps/channels) .
Q. What experimental strategies are effective in delineating the roles of potassium channels vs. Ca²⁺ ATPases in this compound-induced relaxation when cGMP pathways are inhibited?
- Pharmacological Tools :
- Functional Assays : Measure relaxation in tissues pre-treated with ODQ (10 μM) and exposed to high-K⁺ depolarizing solutions (80 mM KCl) to suppress K⁺-dependent effects .
Q. How does this compound’s dual role in AMPK activation and glucose metabolism intersect with its vascular effects?
this compound increases AMPK-α1 activity (1.7-fold) in skeletal muscle, enhancing glycogen synthesis and glucose uptake . To study cross-talk between metabolic and vascular pathways:
- Integrated Models : Use L6 myotubes or vascular smooth muscle cells to measure AMPK phosphorylation (Thr172) alongside NO-mediated relaxation .
- Dose-Response Analysis : Compare low-dose (1–10 μM) vs. high-dose (100 μM) effects to differentiate metabolic vs. vascular signaling thresholds .
Q. Methodological Considerations
Q. What are best practices for standardizing this compound concentrations across cell-based vs. tissue-based studies?
- Cell Studies : Use 1–10 μM for AMPK/glycogen studies (low NO flux avoids cytotoxicity) .
- Tissue Studies : Apply 10–100 μM to achieve submaximal relaxation in pre-contracted arteries .
- Normalization : Express responses as % relaxation relative to phenylephrine-induced contraction (e.g., IC25 values) to account for tissue variability .
Q. How can researchers validate the specificity of this compound’s effects in complex physiological systems?
- Negative Controls : Use NO scavengers (e.g., PTIO, 200 μM) to confirm NO-dependent effects .
- Comparative Pharmacology : Test structurally distinct NO donors (e.g., DETA NONOate, SIN-1) to rule out off-target effects of the spermine backbone .
Q. Data Analysis and Interpretation
Q. What statistical approaches are recommended for analyzing non-linear responses in this compound dose-relaxation curves?
- Curve Fitting : Use sigmoidal dose-response models (e.g., log[inhibitor] vs. response in GraphPad Prism) to calculate EC50/IC50 values .
- Outlier Handling : Exclude data points where phenylephrine-induced contractions exceed 500% baseline (prevents skewed normalization) .
Properties
IUPAC Name |
[3-aminopropyl-[4-(3-aminopropylamino)butyl]amino]-hydroxyimino-oxidoazanium | |
---|---|---|
Details | Computed by Lexichem TK 2.7.0 (PubChem release 2021.05.07) | |
Source | PubChem | |
URL | https://pubchem.ncbi.nlm.nih.gov | |
Description | Data deposited in or computed by PubChem | |
InChI |
InChI=1S/C10H26N6O2/c11-5-3-8-13-7-1-2-9-15(10-4-6-12)16(18)14-17/h13,17H,1-12H2 | |
Details | Computed by InChI 1.0.6 (PubChem release 2021.05.07) | |
Source | PubChem | |
URL | https://pubchem.ncbi.nlm.nih.gov | |
Description | Data deposited in or computed by PubChem | |
InChI Key |
STVYBUMQUAJDER-UHFFFAOYSA-N | |
Details | Computed by InChI 1.0.6 (PubChem release 2021.05.07) | |
Source | PubChem | |
URL | https://pubchem.ncbi.nlm.nih.gov | |
Description | Data deposited in or computed by PubChem | |
Canonical SMILES |
C(CCN(CCCN)[N+](=NO)[O-])CNCCCN | |
Details | Computed by OEChem 2.3.0 (PubChem release 2021.05.07) | |
Source | PubChem | |
URL | https://pubchem.ncbi.nlm.nih.gov | |
Description | Data deposited in or computed by PubChem | |
Molecular Formula |
C10H26N6O2 | |
Details | Computed by PubChem 2.1 (PubChem release 2021.05.07) | |
Source | PubChem | |
URL | https://pubchem.ncbi.nlm.nih.gov | |
Description | Data deposited in or computed by PubChem | |
Molecular Weight |
262.35 g/mol | |
Details | Computed by PubChem 2.1 (PubChem release 2021.05.07) | |
Source | PubChem | |
URL | https://pubchem.ncbi.nlm.nih.gov | |
Description | Data deposited in or computed by PubChem | |
Retrosynthesis Analysis
AI-Powered Synthesis Planning: Our tool employs the Template_relevance Pistachio, Template_relevance Bkms_metabolic, Template_relevance Pistachio_ringbreaker, Template_relevance Reaxys, Template_relevance Reaxys_biocatalysis model, leveraging a vast database of chemical reactions to predict feasible synthetic routes.
One-Step Synthesis Focus: Specifically designed for one-step synthesis, it provides concise and direct routes for your target compounds, streamlining the synthesis process.
Accurate Predictions: Utilizing the extensive PISTACHIO, BKMS_METABOLIC, PISTACHIO_RINGBREAKER, REAXYS, REAXYS_BIOCATALYSIS database, our tool offers high-accuracy predictions, reflecting the latest in chemical research and data.
Strategy Settings
Precursor scoring | Relevance Heuristic |
---|---|
Min. plausibility | 0.01 |
Model | Template_relevance |
Template Set | Pistachio/Bkms_metabolic/Pistachio_ringbreaker/Reaxys/Reaxys_biocatalysis |
Top-N result to add to graph | 6 |
Feasible Synthetic Routes
Disclaimer and Information on In-Vitro Research Products
Please be aware that all articles and product information presented on BenchChem are intended solely for informational purposes. The products available for purchase on BenchChem are specifically designed for in-vitro studies, which are conducted outside of living organisms. In-vitro studies, derived from the Latin term "in glass," involve experiments performed in controlled laboratory settings using cells or tissues. It is important to note that these products are not categorized as medicines or drugs, and they have not received approval from the FDA for the prevention, treatment, or cure of any medical condition, ailment, or disease. We must emphasize that any form of bodily introduction of these products into humans or animals is strictly prohibited by law. It is essential to adhere to these guidelines to ensure compliance with legal and ethical standards in research and experimentation.