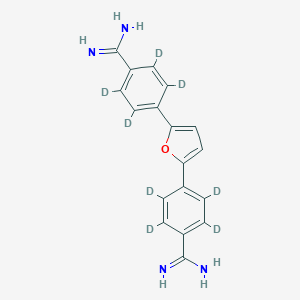
Furamidine-d8
- Click on QUICK INQUIRY to receive a quote from our team of experts.
- With the quality product at a COMPETITIVE price, you can focus more on your research.
Overview
Description
Furamidine-d8 is a synthetic organic compound characterized by the presence of deuterium atoms, which are isotopes of hydrogen
Mechanism of Action
Target of Action
Furamidine-d8 primarily targets Protein Arginine Methyltransferase 1 (PRMT1) . PRMT1 is an essential epigenetic and post-translational regulator in eukaryotic organisms, and its dysregulation is intimately related to multiple types of human diseases, particularly cancer . This compound also inhibits Tyrosyl-DNA Phosphodiesterase 1 (TDP-1) .
Mode of Action
This compound interacts with its targets, leading to significant changes in their activity. It acts as a potent and selective inhibitor for PRMT1 . It’s also a reversible and competitive inhibitor of TDP-1 . The inhibition of TDP-1 by this compound is effective both with single- and double-stranded DNA substrates .
Biochemical Pathways
This compound affects the biochemical pathways associated with its targets. PRMT1 modulates chromatin remodeling by depositing methyl marks on specific arginine residues of nucleosomal histones . Major PRMTs also have a broad spectrum of non-histone substrates that are pertinent to numerous signaling pathways .
Result of Action
The molecular and cellular effects of this compound’s action are significant. It inhibits cell proliferation and reduces the arginine asymmetric dimethylation level in leukemia cancer cells . It also induces apoptosis .
Biochemical Analysis
Biochemical Properties
Furamidine-d8 has been identified as an inhibitor of protein arginine methyltransferase 1 (PRMT1), a key enzyme involved in the methylation of arginine residues on histone and non-histone proteins . This interaction with PRMT1 suggests that this compound plays a role in modulating epigenetic regulation and post-translational modifications .
Cellular Effects
This compound has been shown to have significant effects on various types of cells. For instance, it has been found to inhibit cell proliferation in leukemia cell lines . In glioblastoma stem cells (GSCs), this compound has been shown to effectively inhibit proliferation and tumorsphere formation by inducing cell cycle arrest at the G0/G1 phase and promoting the intrinsic apoptotic pathway .
Molecular Mechanism
The molecular mechanism of this compound involves its interaction with DNA. It has been found to accumulate in the cell nuclei and mitochondria, where it binds to AT sites in the DNA . This binding interaction provides the driving force that permits massive accumulation of this compound in the nuclei .
Metabolic Pathways
This compound, as a PRMT1 inhibitor, is likely involved in the metabolic pathways related to arginine methylation
Transport and Distribution
This compound has been found to accumulate selectively in the cell nuclei . The presence of aromatic rings on the terminal side chain is a limiting factor that restricts the uptake of the compounds in the nuclear compartment .
Subcellular Localization
This compound and its phenyl-substituted analogue accumulate in the cell nuclei and mitochondria, respectively . This suggests that this compound may be directed to these specific compartments or organelles within the cell.
Preparation Methods
The synthesis of Furamidine-d8 involves multiple steps, including the introduction of deuterium atoms. The synthetic route typically starts with the preparation of the furan and benzene rings, followed by the introduction of the carbamimidoyl group. Reaction conditions often involve the use of deuterated reagents and solvents to ensure the incorporation of deuterium atoms. Industrial production methods may involve large-scale synthesis using optimized reaction conditions to achieve high yields and purity.
Chemical Reactions Analysis
This compound undergoes various types of chemical reactions, including:
Oxidation: The compound can be oxidized under specific conditions to form corresponding oxidized products.
Reduction: Reduction reactions can be performed to modify the functional groups present in the compound.
Substitution: The compound can undergo substitution reactions, where one functional group is replaced by another. Common reagents used in these reactions include oxidizing agents like potassium permanganate, reducing agents like sodium borohydride, and various nucleophiles for substitution reactions. The major products formed depend on the specific reaction conditions and reagents used.
Scientific Research Applications
Furamidine-d8 has several scientific research applications:
Chemistry: Used as a labeled compound in studies involving reaction mechanisms and kinetics.
Biology: Employed in metabolic studies to trace biochemical pathways.
Medicine: Investigated for its potential therapeutic effects and as a diagnostic tool.
Industry: Utilized in the development of new materials and chemical processes.
Comparison with Similar Compounds
Compared to other similar compounds, Furamidine-d8 is unique due to the presence of multiple deuterium atoms. Similar compounds include:
4-[5-(4-Carbamimidoylphenyl)furan-2-yl]benzenecarboximidamide: Lacks deuterium atoms.
4-[5-(4-Carbamimidoyl-2,3,5,6-tetradeuteriophenyl)furan-2-yl]benzenecarboximidamide: Contains deuterium atoms only in the phenyl ring.
4-[5-(4-Carbamimidoylphenyl)furan-2-yl]-2,3,5,6-tetradeuteriobenzenecarboximidamide: Contains deuterium atoms only in the benzene ring.
These comparisons highlight the unique isotopic labeling of this compound, which can be advantageous in various research applications.
Properties
IUPAC Name |
4-[5-(4-carbamimidoyl-2,3,5,6-tetradeuteriophenyl)furan-2-yl]-2,3,5,6-tetradeuteriobenzenecarboximidamide |
Source
|
---|---|---|
Source | PubChem | |
URL | https://pubchem.ncbi.nlm.nih.gov | |
Description | Data deposited in or computed by PubChem | |
InChI |
InChI=1S/C18H16N4O/c19-17(20)13-5-1-11(2-6-13)15-9-10-16(23-15)12-3-7-14(8-4-12)18(21)22/h1-10H,(H3,19,20)(H3,21,22)/i1D,2D,3D,4D,5D,6D,7D,8D |
Source
|
Source | PubChem | |
URL | https://pubchem.ncbi.nlm.nih.gov | |
Description | Data deposited in or computed by PubChem | |
InChI Key |
ZJHZBDRZEZEDGB-PGRXLJNUSA-N |
Source
|
Source | PubChem | |
URL | https://pubchem.ncbi.nlm.nih.gov | |
Description | Data deposited in or computed by PubChem | |
Canonical SMILES |
C1=CC(=CC=C1C2=CC=C(O2)C3=CC=C(C=C3)C(=N)N)C(=N)N |
Source
|
Source | PubChem | |
URL | https://pubchem.ncbi.nlm.nih.gov | |
Description | Data deposited in or computed by PubChem | |
Isomeric SMILES |
[2H]C1=C(C(=C(C(=C1C2=CC=C(O2)C3=C(C(=C(C(=C3[2H])[2H])C(=N)N)[2H])[2H])[2H])[2H])C(=N)N)[2H] |
Source
|
Source | PubChem | |
URL | https://pubchem.ncbi.nlm.nih.gov | |
Description | Data deposited in or computed by PubChem | |
Molecular Formula |
C18H16N4O |
Source
|
Source | PubChem | |
URL | https://pubchem.ncbi.nlm.nih.gov | |
Description | Data deposited in or computed by PubChem | |
Molecular Weight |
312.4 g/mol |
Source
|
Source | PubChem | |
URL | https://pubchem.ncbi.nlm.nih.gov | |
Description | Data deposited in or computed by PubChem | |
Disclaimer and Information on In-Vitro Research Products
Please be aware that all articles and product information presented on BenchChem are intended solely for informational purposes. The products available for purchase on BenchChem are specifically designed for in-vitro studies, which are conducted outside of living organisms. In-vitro studies, derived from the Latin term "in glass," involve experiments performed in controlled laboratory settings using cells or tissues. It is important to note that these products are not categorized as medicines or drugs, and they have not received approval from the FDA for the prevention, treatment, or cure of any medical condition, ailment, or disease. We must emphasize that any form of bodily introduction of these products into humans or animals is strictly prohibited by law. It is essential to adhere to these guidelines to ensure compliance with legal and ethical standards in research and experimentation.