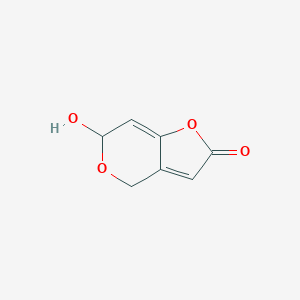
Neopatulin
Overview
Description
Isopatulin is a furopyran and lactone that is (2H-pyran-3(6H)-ylidene)acetic acid which is substituted by hydroxy groups at positions 4 an 6 and in which the hydroxy group at position 4 has condensed with the carboxy group to give the corresponding bicyclic lactone. Also known as neopatulin, it is a mycotoxic substance produced by Aspergillus and Penicillium species. It has a role as a mycotoxin, an antibacterial agent and a Penicillium metabolite. It is a gamma-lactone, a furopyran and a lactol.
Mechanism of Action
Target of Action
Neopatulin, a secondary metabolite produced by numerous fungal species, especially within Aspergillus, Byssochlamys, and Penicillium genera , has been shown to possess immunosuppressive properties . The primary targets of this compound are macrophages , a heterogeneous population of immune cells widely distributed throughout organs and connective tissue . Macrophages play a central role in the immune response, with functions including engulfing and destroying foreign bodies through phagocytosis .
Mode of Action
This compound interacts with its primary targets, the macrophages, and alters their function. It has been shown to encompass cell activation, cytokine release, immune cell function, mitogen-activated protein kinases (MAPKs) activation, nitric oxide production, nuclear factor κ B (NF-κB) activation, phagocytosis, and reactive oxygen species production . These interactions result in changes in the immune response of the host .
Biochemical Pathways
The biosynthetic pathway of this compound consists of approximately 10 steps . The enzyme PatF converts phyllostine into this compound, which is an essential step in patulin biosynthesis . An aldehyde group is produced at the C-5 position and a carboxylic acid group at the C-6 positions, which forms the hemiacetal and lactone bonds found in this compound .
Result of Action
The molecular and cellular effects of this compound’s action are primarily immunosuppressive. It affects humoral and cellular immune response, immune cell function and activation, phagocytosis, nitric oxide and reactive oxygen species production, cytokine release, and nuclear factor-κB and mitogen-activated protein kinases activation .
Action Environment
Environmental factors can influence the action, efficacy, and stability of this compound. Several factors affect the colonization and production of mycotoxins by fungi, including pH, temperature, humidity, and substrate nutrition . These factors can potentially influence the production and action of this compound.
Biochemical Analysis
Biochemical Properties
The enzyme PatF converts phyllostine into Neopatulin, which is an essential step in patulin biosynthesis . An aldehyde group is produced at the C-5 position and a carboxylic acid group at the C-6 positions which forms the hemiacetal and lactone bonds found in this compound .
Cellular Effects
As a mycotoxin, it is known to have toxic effects on cells, particularly in the gastrointestinal tract .
Molecular Mechanism
It is known that the biosynthetic pathway consists of approximately 10 steps, as suggested by biochemical studies .
Properties
IUPAC Name |
6-hydroxy-4,6-dihydrofuro[3,2-c]pyran-2-one | |
---|---|---|
Source | PubChem | |
URL | https://pubchem.ncbi.nlm.nih.gov | |
Description | Data deposited in or computed by PubChem | |
InChI |
InChI=1S/C7H6O4/c8-6-2-5-4(3-10-6)1-7(9)11-5/h1-2,6,8H,3H2 | |
Source | PubChem | |
URL | https://pubchem.ncbi.nlm.nih.gov | |
Description | Data deposited in or computed by PubChem | |
InChI Key |
ZGBMSNKHUHZKEP-UHFFFAOYSA-N | |
Source | PubChem | |
URL | https://pubchem.ncbi.nlm.nih.gov | |
Description | Data deposited in or computed by PubChem | |
Canonical SMILES |
C1C2=CC(=O)OC2=CC(O1)O | |
Source | PubChem | |
URL | https://pubchem.ncbi.nlm.nih.gov | |
Description | Data deposited in or computed by PubChem | |
Molecular Formula |
C7H6O4 | |
Source | PubChem | |
URL | https://pubchem.ncbi.nlm.nih.gov | |
Description | Data deposited in or computed by PubChem | |
DSSTOX Substance ID |
DTXSID60990610 | |
Record name | 6-Hydroxy-4H-furo[3,2-c]pyran-2(6H)-one | |
Source | EPA DSSTox | |
URL | https://comptox.epa.gov/dashboard/DTXSID60990610 | |
Description | DSSTox provides a high quality public chemistry resource for supporting improved predictive toxicology. | |
Molecular Weight |
154.12 g/mol | |
Source | PubChem | |
URL | https://pubchem.ncbi.nlm.nih.gov | |
Description | Data deposited in or computed by PubChem | |
CAS No. |
70402-10-7 | |
Record name | Neopatulin | |
Source | ChemIDplus | |
URL | https://pubchem.ncbi.nlm.nih.gov/substance/?source=chemidplus&sourceid=0070402107 | |
Description | ChemIDplus is a free, web search system that provides access to the structure and nomenclature authority files used for the identification of chemical substances cited in National Library of Medicine (NLM) databases, including the TOXNET system. | |
Record name | 6-Hydroxy-4H-furo[3,2-c]pyran-2(6H)-one | |
Source | EPA DSSTox | |
URL | https://comptox.epa.gov/dashboard/DTXSID60990610 | |
Description | DSSTox provides a high quality public chemistry resource for supporting improved predictive toxicology. | |
Record name | NEOPATULIN | |
Source | FDA Global Substance Registration System (GSRS) | |
URL | https://gsrs.ncats.nih.gov/ginas/app/beta/substances/45ZSX3D9J5 | |
Description | The FDA Global Substance Registration System (GSRS) enables the efficient and accurate exchange of information on what substances are in regulated products. Instead of relying on names, which vary across regulatory domains, countries, and regions, the GSRS knowledge base makes it possible for substances to be defined by standardized, scientific descriptions. | |
Explanation | Unless otherwise noted, the contents of the FDA website (www.fda.gov), both text and graphics, are not copyrighted. They are in the public domain and may be republished, reprinted and otherwise used freely by anyone without the need to obtain permission from FDA. Credit to the U.S. Food and Drug Administration as the source is appreciated but not required. | |
Retrosynthesis Analysis
AI-Powered Synthesis Planning: Our tool employs the Template_relevance Pistachio, Template_relevance Bkms_metabolic, Template_relevance Pistachio_ringbreaker, Template_relevance Reaxys, Template_relevance Reaxys_biocatalysis model, leveraging a vast database of chemical reactions to predict feasible synthetic routes.
One-Step Synthesis Focus: Specifically designed for one-step synthesis, it provides concise and direct routes for your target compounds, streamlining the synthesis process.
Accurate Predictions: Utilizing the extensive PISTACHIO, BKMS_METABOLIC, PISTACHIO_RINGBREAKER, REAXYS, REAXYS_BIOCATALYSIS database, our tool offers high-accuracy predictions, reflecting the latest in chemical research and data.
Strategy Settings
Precursor scoring | Relevance Heuristic |
---|---|
Min. plausibility | 0.01 |
Model | Template_relevance |
Template Set | Pistachio/Bkms_metabolic/Pistachio_ringbreaker/Reaxys/Reaxys_biocatalysis |
Top-N result to add to graph | 6 |
Feasible Synthetic Routes
Q1: What is the significance of neopatulin in relation to other compounds like patulin?
A1: this compound is considered a biogenetic precursor to the mycotoxin patulin [, ]. This means that in the natural biosynthetic pathway, this compound is formed as an intermediate step before being further converted into patulin. Research suggests that both compounds are produced by certain species of Penicillium and Aspergillus fungi [].
Q2: Could you elaborate on the biosynthesis of this compound and patulin?
A2: Research has shed light on the enzymatic processes involved in the biosynthesis of these compounds. Studies using extracts from Penicillium patulum identified an enzyme capable of epoxidizing toluquinol and gentisyl alcohol []. The epoxidation of gentisyl alcohol leads to the formation of phyllostine, a known intermediate in patulin biosynthesis []. Further enzymatic activity converts phyllostine into this compound []. While the exact enzymatic steps from this compound to patulin require further investigation, the epoxidation process appears to be a key mechanism in their biosynthesis.
Q3: Are there efficient methods available for synthesizing this compound in a laboratory setting?
A3: Yes, several research groups have successfully developed total syntheses for this compound [, , , ]. These synthetic routes provide alternative ways to obtain this compound for further research, apart from relying solely on fungal cultures.
Q4: Has there been any computational work done to study this compound?
A4: Density Functional Theory (DFT) has been employed to compare the structural and electronic properties of this compound with related compounds like patulin and ascladiol []. Such computational studies can offer insights into the reactivity, stability, and potential interactions of this compound.
Q5: What is the current understanding of the structure of this compound?
A5: While the provided abstracts do not explicitly detail the molecular formula, weight, or spectroscopic data of this compound, they consistently refer to it as a "ylidenebutenolide mycotoxin" []. This classification points to a specific structural motif within the molecule. For precise structural characterization, referring to the complete research papers would be necessary.
Disclaimer and Information on In-Vitro Research Products
Please be aware that all articles and product information presented on BenchChem are intended solely for informational purposes. The products available for purchase on BenchChem are specifically designed for in-vitro studies, which are conducted outside of living organisms. In-vitro studies, derived from the Latin term "in glass," involve experiments performed in controlled laboratory settings using cells or tissues. It is important to note that these products are not categorized as medicines or drugs, and they have not received approval from the FDA for the prevention, treatment, or cure of any medical condition, ailment, or disease. We must emphasize that any form of bodily introduction of these products into humans or animals is strictly prohibited by law. It is essential to adhere to these guidelines to ensure compliance with legal and ethical standards in research and experimentation.