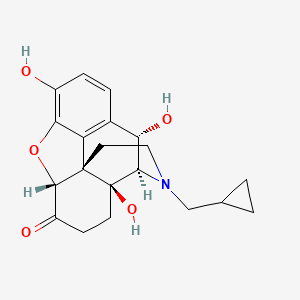
10Alpha-Hydroxy Naltrexone
- Click on QUICK INQUIRY to receive a quote from our team of experts.
- With the quality product at a COMPETITIVE price, you can focus more on your research.
Overview
Description
10Alpha-Hydroxy Naltrexone is a derivative of naltrexone, an opioid receptor antagonist commonly used in the treatment of opioid and alcohol dependence. This compound is characterized by the presence of a hydroxyl group at the 10-alpha position, which distinguishes it from its parent compound, naltrexone .
Preparation Methods
Synthetic Routes and Reaction Conditions: The synthesis of 10Alpha-Hydroxy Naltrexone typically involves the hydroxylation of naltrexone. One common method is the catalytic enantioselective Sharpless dihydroxylation, which introduces the stereogenic centers necessary for the compound . The process involves the use of osmium tetroxide (OsO4) and a chiral ligand, followed by the reduction of the intermediate with sodium bisulfite.
Industrial Production Methods: Industrial production of this compound may involve the use of large-scale reactors and continuous flow systems to ensure consistent quality and yield. The reaction conditions are optimized to maintain the integrity of the hydroxyl group and prevent degradation of the compound .
Chemical Reactions Analysis
Types of Reactions: 10Alpha-Hydroxy Naltrexone undergoes various chemical reactions, including:
Oxidation: The hydroxyl group can be oxidized to form a ketone or aldehyde.
Reduction: The compound can be reduced to remove the hydroxyl group, reverting to naltrexone.
Substitution: The hydroxyl group can be substituted with other functional groups, such as halogens or alkyl groups.
Common Reagents and Conditions:
Oxidation: Reagents like potassium permanganate (KMnO4) or chromium trioxide (CrO3) are commonly used.
Reduction: Sodium borohydride (NaBH4) or lithium aluminum hydride (LiAlH4) are typical reducing agents.
Substitution: Halogenating agents like thionyl chloride (SOCl2) or phosphorus tribromide (PBr3) are used for substitution reactions.
Major Products:
Oxidation: Formation of 10-keto naltrexone.
Reduction: Reversion to naltrexone.
Substitution: Formation of 10-halogenated naltrexone derivatives.
Scientific Research Applications
10Alpha-Hydroxy Naltrexone has a wide range of applications in scientific research:
Mechanism of Action
10Alpha-Hydroxy Naltrexone exerts its effects by binding to opioid receptors, primarily the mu-opioid receptor. This binding blocks the euphoric effects of opioids and reduces cravings for alcohol. The compound acts as a competitive antagonist, preventing endogenous opioids from activating the receptor . The hydroxyl group at the 10-alpha position may enhance its binding affinity and selectivity for the receptor .
Comparison with Similar Compounds
Naltrexone: The parent compound, used for opioid and alcohol dependence treatment.
Naloxone: Another opioid antagonist, used primarily for the reversal of opioid overdose.
Nalmefene: Similar to naltrexone, used for the treatment of alcohol dependence.
Uniqueness: 10Alpha-Hydroxy Naltrexone is unique due to the presence of the hydroxyl group at the 10-alpha position, which may confer different pharmacokinetic and pharmacodynamic properties compared to its parent compound and other similar compounds . This structural modification can potentially enhance its efficacy and reduce side effects .
Properties
Molecular Formula |
C20H23NO5 |
---|---|
Molecular Weight |
357.4 g/mol |
IUPAC Name |
(4R,4aS,7aR,12bS,13S)-3-(cyclopropylmethyl)-4a,9,13-trihydroxy-2,4,5,6,7a,13-hexahydro-1H-4,12-methanobenzofuro[3,2-e]isoquinolin-7-one |
InChI |
InChI=1S/C20H23NO5/c22-12-4-3-11-14-16(12)26-18-13(23)5-6-20(25)17(15(11)24)21(9-10-1-2-10)8-7-19(14,18)20/h3-4,10,15,17-18,22,24-25H,1-2,5-9H2/t15-,17+,18-,19-,20+/m0/s1 |
InChI Key |
SPLLFLBLAXVPPQ-AXDKOMKPSA-N |
Isomeric SMILES |
C1CC1CN2CC[C@]34[C@@H]5C(=O)CC[C@]3([C@H]2[C@H](C6=C4C(=C(C=C6)O)O5)O)O |
Canonical SMILES |
C1CC1CN2CCC34C5C(=O)CCC3(C2C(C6=C4C(=C(C=C6)O)O5)O)O |
Origin of Product |
United States |
Disclaimer and Information on In-Vitro Research Products
Please be aware that all articles and product information presented on BenchChem are intended solely for informational purposes. The products available for purchase on BenchChem are specifically designed for in-vitro studies, which are conducted outside of living organisms. In-vitro studies, derived from the Latin term "in glass," involve experiments performed in controlled laboratory settings using cells or tissues. It is important to note that these products are not categorized as medicines or drugs, and they have not received approval from the FDA for the prevention, treatment, or cure of any medical condition, ailment, or disease. We must emphasize that any form of bodily introduction of these products into humans or animals is strictly prohibited by law. It is essential to adhere to these guidelines to ensure compliance with legal and ethical standards in research and experimentation.