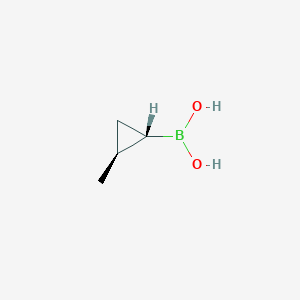
((1S,2S)-2-Methylcyclopropyl)boronic acid
- Click on QUICK INQUIRY to receive a quote from our team of experts.
- With the quality product at a COMPETITIVE price, you can focus more on your research.
Overview
Description
((1S,2S)-2-Methylcyclopropyl)boronic acid is a chiral cyclopropane-containing boronic acid with the molecular formula C₄H₉BO₂ and a molecular weight of 99.92 g/mol . This compound is characterized by its stereospecific (1S,2S) configuration, which imparts distinct reactivity in cross-coupling reactions such as Suzuki-Miyaura couplings. Boronic acids of this class are critical in medicinal chemistry and materials science due to their ability to form stable covalent bonds with diols and transition metals. Safety protocols for handling this compound include precautions against ignition sources (P210) and mandates for pre-use safety reviews (P201, P202) .
Preparation Methods
Synthetic Routes and Reaction Conditions:
Hydroboration of Alkenes: One common method for preparing ((1S,2S)-2-Methylcyclopropyl)boronic acid involves the hydroboration of alkenes. This reaction typically uses borane (BH3) or its derivatives as the boron source. The reaction is carried out under an inert atmosphere, such as nitrogen or argon, to prevent oxidation.
Asymmetric Synthesis: Asymmetric synthesis can be employed to obtain the desired enantiomer of this compound. Chiral catalysts or chiral auxiliaries are used to induce chirality in the product.
Industrial Production Methods: Industrial production of this compound may involve large-scale hydroboration processes with optimized reaction conditions to maximize yield and purity. The use of continuous flow reactors and advanced purification techniques can enhance the efficiency of production.
Chemical Reactions Analysis
Types of Reactions:
Oxidation: ((1S,2S)-2-Methylcyclopropyl)boronic acid can undergo oxidation reactions to form the corresponding alcohol or ketone. Common oxidizing agents include hydrogen peroxide (H2O2) and sodium perborate.
Reduction: Reduction of this compound can yield the corresponding alkane. Reducing agents such as lithium aluminum hydride (LiAlH4) or sodium borohydride (NaBH4) are typically used.
Substitution: This compound can participate in substitution reactions where the boronic acid group is replaced by other functional groups. For example, it can react with halides in the presence of a palladium catalyst to form carbon-carbon bonds.
Common Reagents and Conditions:
Oxidation: Hydrogen peroxide, sodium perborate, and other oxidizing agents.
Reduction: Lithium aluminum hydride, sodium borohydride, and other reducing agents.
Substitution: Palladium catalysts, halides, and other electrophiles.
Major Products:
Oxidation: Alcohols, ketones.
Reduction: Alkanes.
Substitution: Various substituted cyclopropyl derivatives.
Scientific Research Applications
Chemistry: ((1S,2S)-2-Methylcyclopropyl)boronic acid is used as a building block in organic synthesis. It can be employed in cross-coupling reactions, such as Suzuki-Miyaura coupling, to form carbon-carbon bonds.
Biology: In biological research, this compound can be used to study enzyme mechanisms and as a probe for boron-containing biomolecules.
Medicine: this compound has potential applications in medicinal chemistry. It can be used to design boron-containing drugs with improved pharmacokinetic properties.
Industry: In the industrial sector, this compound can be used in the synthesis of advanced materials and as a catalyst in various chemical processes.
Mechanism of Action
The mechanism of action of ((1S,2S)-2-Methylcyclopropyl)boronic acid involves its ability to form reversible covalent bonds with nucleophiles. The boronic acid group can interact with hydroxyl groups, amines, and other nucleophiles to form boronate esters or complexes. These interactions are crucial in its role as a catalyst and in biological systems.
Comparison with Similar Compounds
Stereoisomeric Variants
The stereochemical configuration of cyclopropylboronic acids significantly influences their physical properties and synthetic utility. Key stereoisomers include:
((1R,2R)-2-Methylcyclopropyl)boronic Acid
- Synthesis : Produced via chiral auxiliaries like (4S,5S)-tetramethyl-D-tartaric acid diamide ester, yielding enantiomeric ratios (er) of 94:6 .
- Optical Rotation : Expected to exhibit an optical rotation ([α]D) opposite to the (1S,2S) enantiomer, analogous to sulfone derivatives reported in literature .
((1R,2S)-2-Methylcyclopropyl)boronic Acid
Structural Analogs and Derivatives
((1R,2S)-2-(Methoxycarbonyl)-2-methylcyclopropyl)boronic Acid
- Molecular Formula : C₆H₁₁BO₄ (MW: 157.96 g/mol) .
- Purity : >99%, with a defined Smile code (C[C@@]1(C(OC)=O)C@HC1 ) indicating stereochemical precision .
- Applications : The methoxycarbonyl group enhances stability and modifies electronic properties for targeted reactivity.
αR-(3-Bromopropyl)boronic Acid Pinanediol Ester
- Molecular Formula: C₁₄H₂₅BBrNO₂ (MW: 330.07 g/mol) .
- Commercial Data : Priced at $2,000/0.1 g , reflecting the complexity of its pinanediol-protected structure .
Data Table: Comparative Analysis
Biological Activity
((1S,2S)-2-Methylcyclopropyl)boronic acid is a boronic acid derivative that has garnered attention for its potential biological activities, particularly in the fields of medicinal chemistry and biochemistry. This article delves into its biological activity, focusing on its inhibition profiles against various enzymes, particularly β-lactamases, and its implications in drug development.
- Molecular Formula : C4H9BO2
- CAS Number : 124636913
- Structural Characteristics : The compound features a cyclopropyl group substituted with a boronic acid functional group, which is critical for its biological interactions.
Boronic acids, including this compound, primarily function as inhibitors of serine and metallo-β-lactamases (BLs). These enzymes are responsible for antibiotic resistance in various bacterial strains. The mechanism involves the formation of a covalent bond with the active site serine residue of the enzyme, resulting in the inhibition of its activity.
Inhibition Profiles
Recent studies have characterized the inhibition profiles of several boronic acids against clinically relevant β-lactamases. For instance:
- Inhibition of Serine β-Lactamases : IC50 values were determined for various compounds, indicating that this compound exhibits significant inhibitory activity against AmpC β-lactamase with an IC50 value of approximately 38 µM. Other compounds showed weaker inhibition with IC50 values ranging from 60 µM to 132 µM .
- Metallo-β-Lactamases (MBLs) : The compound also demonstrated activity against MBLs such as NDM-1 and VIM-2. The IC50 values for these enzymes were reported to be around 36 µM and 32 µM respectively, suggesting a promising profile for combating antibiotic resistance .
Comparative Analysis of Inhibition Activities
Compound | Target Enzyme | IC50 (µM) |
---|---|---|
This compound | AmpC β-lactamase | 38 |
NDM-1 | 36 | |
VIM-2 | 32 | |
Other Boronic Acids | Various Serine BLs | 60 - 132 |
Study on Boronic Acids in Medicinal Chemistry
A comprehensive review highlighted the diverse biological activities of boronic acids, including their anticancer and antibacterial properties. The study emphasized that derivatives like this compound could serve as effective scaffolds for developing new therapeutic agents against resistant bacterial strains .
Clinical Implications
The potential application of this compound in combination therapies has been explored. For example, it has been studied alongside bortezomib in treating advanced lymphomas to overcome drug resistance mechanisms associated with pro-apoptotic proteins .
Q & A
Basic Research Questions
Q. What synthetic methodologies are recommended for achieving high enantiomeric purity in ((1S,2S)-2-Methylcyclopropyl)boronic acid?
Enantioselective synthesis can be achieved via asymmetric cyclopropanation using chiral catalysts (e.g., transition-metal complexes with bisoxazoline ligands) or enzymatic resolution. For cyclopropane derivatives, stereochemical control often relies on optimizing reaction conditions (temperature, solvent polarity) to favor the desired (1S,2S) diastereomer. Post-synthesis purification via chiral HPLC or crystallization with resolving agents (e.g., tartaric acid derivatives) can further enhance enantiomeric excess .
Q. Which analytical techniques are critical for characterizing this compound?
- NMR Spectroscopy : 1H and 11B NMR confirm structural integrity and boron environment.
- LC-MS : Validates molecular weight and detects impurities (e.g., hydrolysis products) using reverse-phase columns with formic acid/ACN gradients .
- Thermogravimetric Analysis (TGA) : Assesses thermal stability and degradation thresholds under controlled atmospheres (e.g., N2 or O2) .
- X-ray Crystallography : Resolves absolute stereochemistry and boron coordination geometry .
Q. How should this compound be stored to prevent degradation?
Store at -20°C in airtight, light-resistant containers under inert gas (argon or nitrogen). Use desiccants to minimize moisture uptake, as boronic acids are prone to hydrolysis. For aqueous work, prepare solutions fresh or stabilize with glycerol (10–20% v/v) to slow decomposition .
Advanced Research Questions
Q. How does the stereochemistry of this compound influence its reactivity in Suzuki-Miyaura cross-coupling reactions?
The (1S,2S) configuration introduces steric hindrance near the boron atom, which can slow transmetallation but improve selectivity in aryl-aryl bond formation. Computational studies (DFT calculations) suggest that the methyl group at C2 restricts rotation, favoring planar transition states. Experimentally, compare coupling yields with stereoisomers under identical conditions (Pd catalyst, base, solvent) to quantify stereoelectronic effects .
Q. What strategies mitigate hydrolysis of this compound in aqueous reaction systems?
- Protecting Groups : Convert the boronic acid to its pinacol ester, which is hydrolytically stable. Deprotection can be achieved with mild acids (e.g., HCl in THF) .
- pH Control : Maintain reaction pH between 7.5–8.5 using HEPES buffer to balance boronate anion formation and protonation-induced hydrolysis .
- Co-solvents : Use water-miscible organic solvents (e.g., DMSO, dioxane) to reduce water activity .
Q. How can contradictions in catalytic activity data for this compound be resolved?
- Purity Assessment : Verify enantiomeric excess via chiral HPLC and quantify trace impurities (e.g., boroxines) by 11B NMR .
- Reaction Parameter Screening : Systematically vary Pd catalyst loading (0.5–5 mol%), ligand type (e.g., SPhos vs. XPhos), and base (K2CO3 vs. CsF) to identify optimal conditions .
- Side-Reaction Profiling : Monitor protodeboronation byproducts using LC-MS and adjust reaction time/temperature to minimize degradation .
Q. What computational approaches model the interaction of this compound with biological targets?
- Molecular Docking : Use AutoDock Vina to predict binding poses with proteases (e.g., SARS-CoV-2 Mpro), focusing on boron’s interaction with catalytic serine or cysteine residues .
- Molecular Dynamics (MD) Simulations : Simulate solvent effects (explicit water models) to assess binding stability over 100-ns trajectories. Compare with non-boron analogs to validate boronic acid-specific interactions .
Q. Data Contradiction Analysis
Q. Why do studies report conflicting thermal stability thresholds for this compound?
Discrepancies arise from differing experimental setups:
- TGA Heating Rates : Faster rates (e.g., 20°C/min) may overlook gradual decomposition observed at slower rates (5°C/min) .
- Atmosphere Effects : Oxidative (air) vs. inert (N2) atmospheres alter degradation pathways. For accurate comparisons, standardize conditions to inert gas with ≤1% O2 .
Q. How do functional group modifications on the cyclopropane ring affect the compound’s bioactivity?
Structure-activity relationship (SAR) studies show:
- Methyl at C2 : Enhances metabolic stability but reduces solubility.
- Electron-Withdrawing Groups (e.g., -F) : Increase boron Lewis acidity, improving protease inhibition (e.g., IC50 values for viral proteases) . Tabulate bioactivity data against substituent type and position to identify pharmacophore requirements .
Q. Methodological Tables
Table 1. Comparison of Analytical Techniques for Boronic Acid Characterization
Technique | Key Parameters | Application Example | Reference |
---|---|---|---|
Chiral HPLC | Column: Chiralpak IB-3; Mobile phase: Hexane/IPA (90:10) | Enantiomeric excess determination | |
11B NMR | Frequency: 128 MHz; Solvent: CDCl3 | Detection of boroxine impurities | |
TGA | Heating rate: 5°C/min; Atmosphere: N2 | Thermal decomposition onset (~200°C) |
Table 2. Optimized Conditions for Suzuki-Miyaura Coupling
Parameter | Optimal Value | Impact on Yield |
---|---|---|
Catalyst | Pd(OAc)2/SPhos (2 mol%) | Enhances turnover frequency (TOF) |
Base | Cs2CO3 | Stabilizes boronate intermediate |
Solvent | Dioxane/H2O (4:1) | Balances solubility and reactivity |
Properties
Molecular Formula |
C4H9BO2 |
---|---|
Molecular Weight |
99.93 g/mol |
IUPAC Name |
[(1S,2S)-2-methylcyclopropyl]boronic acid |
InChI |
InChI=1S/C4H9BO2/c1-3-2-4(3)5(6)7/h3-4,6-7H,2H2,1H3/t3-,4-/m0/s1 |
InChI Key |
DYSMDSGBCAQRLB-IMJSIDKUSA-N |
Isomeric SMILES |
B([C@H]1C[C@@H]1C)(O)O |
Canonical SMILES |
B(C1CC1C)(O)O |
Origin of Product |
United States |
Disclaimer and Information on In-Vitro Research Products
Please be aware that all articles and product information presented on BenchChem are intended solely for informational purposes. The products available for purchase on BenchChem are specifically designed for in-vitro studies, which are conducted outside of living organisms. In-vitro studies, derived from the Latin term "in glass," involve experiments performed in controlled laboratory settings using cells or tissues. It is important to note that these products are not categorized as medicines or drugs, and they have not received approval from the FDA for the prevention, treatment, or cure of any medical condition, ailment, or disease. We must emphasize that any form of bodily introduction of these products into humans or animals is strictly prohibited by law. It is essential to adhere to these guidelines to ensure compliance with legal and ethical standards in research and experimentation.