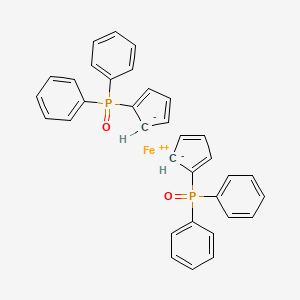
1,1'-Bis(diphenylphosphino)ferrocene dioxide
Overview
Description
1,1'-Bis(diphenylphosphino)ferrocene (dppf) is a bidentate ligand featuring two diphenylphosphine groups attached to a ferrocene backbone. Its oxidized form, 1,1'-Bis(diphenylphosphino)ferrocene dioxide, retains the ferrocene core but with oxidized phosphorus atoms (P=O), altering its electronic and steric properties. This compound is widely used in catalysis, particularly in transition metal complexes for reactions such as cross-coupling and CO₂ activation. Its rigid ferrocene backbone provides geometric stability, while the phosphine oxide groups enhance solubility in polar solvents .
Preparation Methods
Preparation of 1,1'-Bis(diphenylphosphino)ferrocene (dppf) Precursor
The synthesis of 1,1'-bis(diphenylphosphino)ferrocene (dppf) is a critical step before oxidation to the dioxide. The most established method involves the dilithiation of ferrocene followed by reaction with chlorodiphenylphosphine:
- Dilithiation of Ferrocene : Ferrocene is treated with n-butyllithium in the presence of tetramethylethylenediamine (TMEDA) to generate dilithioferrocene intermediates.
- Phosphination : The dilithioferrocene reacts with chlorodiphenylphosphine (ClPPh2) to yield dppf and lithium chloride as a byproduct.
The reaction scheme is:
$$
\text{Fe(C}5\text{H}4\text{Li)}2 + 2 \text{ClPPh}2 \rightarrow \text{Fe(C}5\text{H}4\text{PPh}2)2 + 2 \text{LiCl}
$$
This method is efficient and widely used due to the ease of dilithiation and the availability of reagents.
An alternative reported synthetic route involves the reduction of 1,1'-bis(diphenyloxyphosphino)ferrocene using tributylphosphine and iodine in a tetrahydrofuran/acetonitrile solvent mixture under inert atmosphere. This method achieves high yields (~94%) and involves mild reaction conditions at room temperature, followed by aqueous quenching and recrystallization from ethanol.
Table 1: Summary of dppf Preparation Methods
Oxidation to 1,1'-Bis(diphenylphosphino)ferrocene Dioxide
The preparation of the dioxide form involves the controlled oxidation of the phosphorus atoms in dppf to phosphine oxides. Although detailed procedures specifically for the dioxide are less frequently reported, typical oxidation methods for phosphines apply, such as:
- Use of Oxygen or Peroxides : Mild oxidants like hydrogen peroxide or m-chloroperbenzoic acid (m-CPBA) can selectively oxidize the phosphorus centers to phosphine oxides.
- Controlled Reaction Conditions : Oxidation is carried out under controlled temperature and solvent conditions to avoid overoxidation or degradation of the ferrocene core.
The oxidation converts the P(III) centers in dppf to P(V) centers, resulting in the dioxide.
Research Findings on Oxidation
Studies on related diphosphine ligands indicate that oxidation proceeds smoothly with peracids in solvents such as dichloromethane or chloroform, yielding crystalline phosphine oxide products. The resulting dioxide retains the ferrocene backbone and exhibits distinct spectroscopic signatures (e.g., ^31P NMR shifts and IR P=O stretching bands).
Characterization and Purification
The product this compound is typically isolated as a crystalline solid, often yellow to orange in color, with melting points around 180-182 °C (decomposition). It is air-sensitive and requires storage under inert atmosphere at low temperature (2-8 °C) to maintain stability.
Purification is commonly achieved by recrystallization from ethanol or similar solvents after solvent removal under vacuum. Washing with aqueous sodium bicarbonate and drying over anhydrous sodium sulfate are standard steps to remove impurities.
Summary Table: Preparation Workflow for this compound
Step | Description | Conditions/Notes |
---|---|---|
1. Dilithiation | Ferrocene + n-butyllithium + TMEDA | Inert atmosphere, low temperature |
2. Phosphination | Dilithioferrocene + chlorodiphenylphosphine | Room temperature, inert atmosphere |
3. Isolation of dppf | Workup with water, extraction, drying, recrystallization | Ethanol recrystallization for purity |
4. Oxidation | dppf + oxidant (e.g., H2O2 or m-CPBA) | Controlled temperature, solvent (CH2Cl2 or CHCl3) |
5. Purification of dioxide | Crystallization, washing, drying | Store under inert atmosphere, low temperature |
Research and Industrial Relevance
The preparation methods for this compound are crucial for producing a ligand with modified electronic and steric properties compared to the parent dppf. This dioxide ligand finds applications in catalysis where phosphine oxides provide different coordination environments.
The literature emphasizes:
- High-yield, mild-condition syntheses for the precursor dppf.
- Selective oxidation methods preserving the ferrocene backbone.
- The importance of inert atmosphere and dry solvents to prevent degradation.
These methods are supported by patent literature and peer-reviewed studies, ensuring reproducibility and scalability for research and industrial applications.
Chemical Reactions Analysis
Types of Reactions
1,1’-bis(diphenylphosphino)ferrocene undergoes various types of reactions, primarily forming metal complexes. It is commonly used in palladium-catalyzed coupling reactions .
Common Reagents and Conditions
Palladium Dichloride: Used to form the palladium derivative (dppf)PdCl₂, which is popular for palladium-catalyzed coupling reactions.
Acetonitrile or Benzonitrile: Used as adducts in the formation of palladium complexes.
Major Products
The major products formed from these reactions include metal complexes such as (dppf)PdCl₂, which are used in various catalytic processes .
Scientific Research Applications
1,1’-bis(diphenylphosphino)ferrocene is extensively used in scientific research due to its versatility as a ligand. Some of its applications include :
Chemistry: Used in homogeneous catalysis, particularly in palladium-catalyzed coupling reactions.
Biology: Employed in the synthesis of coordination compounds.
Medicine: Investigated for its potential in drug development.
Industry: Utilized in the production of functional materials such as tailored dyes and OLED components.
Mechanism of Action
The mechanism of action of 1,1’-bis(diphenylphosphino)ferrocene involves its role as a ligand in catalytic processes. The ferrocene core imparts fine control to catalytic carbon-carbon and carbon-heteroatom coupling reactions, facilitating the formation of various functional macromolecules .
Comparison with Similar Compounds
Comparison with Similar Organophosphorus Ligands
The following compounds are structurally or functionally related to dppf dioxide and are compared based on ligand design, catalytic performance, and physicochemical properties.
Structural Analogues with Modified Phosphine Substituents
Key Observations:
- Steric Effects : Alkyl-substituted variants (e.g., PCy₂, P(t-Bu)₂) exhibit greater steric hindrance than dppf, which can hinder substrate binding but enhance selectivity in crowded catalytic environments .
- Electronic Effects : Aryl phosphines (e.g., PPh₂ in dppf) offer stronger electron-donating capabilities compared to alkyl phosphines, favoring oxidative addition steps in catalysis .
Catalytic Performance in CO₂ Activation
A comparative study of nickel catalysts with different ligands revealed the following (Table 2):
Analysis:
- Energy Barriers : Despite a slightly higher activation energy (ΔG‡ = 22.1 kcal/mol for dppf vs. 20.5 kcal/mol for PCy₃), dppf-based catalysts exhibit theoretical advantages due to favorable orbital interactions .
- Yield Discrepancy : The lower experimental yield of dppf (45% vs. 68% for PCy₃) is attributed to weaker CO₂ capture efficiency, highlighting the trade-off between electronic optimization and practical substrate binding .
Biological Activity
1,1'-Bis(diphenylphosphino)ferrocene dioxide (dppfO₂) is a phosphine oxide derivative of bis(diphenylphosphino)ferrocene (dppf), a well-known ligand in coordination chemistry. This compound has garnered attention not only for its chemical properties but also for its potential biological activities. This article explores the biological activity of dppfO₂, highlighting its mechanisms of action, interactions with biological targets, and relevant case studies.
Chemical Structure
The structure of this compound can be represented as follows:
The biological activity of dppfO₂ primarily stems from its ability to coordinate with metal ions and form complexes that can interact with various biological targets. The following mechanisms have been identified:
- Metal Coordination : dppfO₂ can form stable complexes with transition metals such as palladium and platinum, which may enhance their catalytic properties in biological systems .
- Reactive Oxygen Species (ROS) Generation : The oxidation state of the ferrocene moiety allows dppfO₂ to participate in redox reactions, potentially leading to the generation of ROS that can affect cellular signaling pathways .
- Ligand Properties : As a phosphine oxide, dppfO₂ can act as a ligand in enzyme inhibition or modulation, impacting various biochemical pathways .
Anticancer Properties
Recent studies have investigated the anticancer potential of dppfO₂ through its metal complexes. For instance, palladium complexes derived from dppfO₂ exhibited significant cytotoxicity against various cancer cell lines. The mechanism involves the induction of apoptosis via ROS generation and disruption of mitochondrial function .
Antimicrobial Activity
Research has indicated that dppfO₂ and its metal complexes possess antimicrobial properties. The coordination of metals enhances the compound's ability to disrupt bacterial cell membranes or interfere with microbial metabolic pathways .
Enzyme Inhibition
dppfO₂ has been studied for its ability to inhibit specific enzymes involved in cancer and bacterial metabolism. For example, it has shown promise in inhibiting proteases and kinases, which are critical for cell proliferation and survival in cancer cells .
Data Tables
Q & A
Basic Research Questions
Q. What synthetic methodologies are commonly employed to prepare 1,1'-bis(diphenylphosphino)ferrocene dioxide (DppfO₂), and how can purity be verified?
- Methodology : DppfO₂ is typically synthesized by oxidizing 1,1'-bis(diphenylphosphino)ferrocene (Dppf) using mild oxidizing agents like hydrogen peroxide or dimethyl sulfoxide (DMSO). The reaction progress is monitored via ³¹P NMR spectroscopy to confirm the conversion of P(III) to P(V) oxidation states, evidenced by a shift in resonance peaks from ~–20 ppm (Dppf) to ~35 ppm (DppfO₂) . Post-synthesis, purification via recrystallization or column chromatography ensures high purity (>98%), with final characterization using elemental analysis, mass spectrometry, and IR spectroscopy to confirm the absence of unreacted Dppf or byproducts .
Q. Which spectroscopic and crystallographic techniques are critical for characterizing DppfO₂ and its metal complexes?
- Techniques :
- X-ray crystallography : Resolves the geometry of DppfO₂-metal complexes (e.g., bond angles, ligand coordination mode). For example, in [Fe₂(CO)₅(κ¹-DppfO)(µ-benzenedithiolate)], crystallography confirmed the bidentate coordination of DppfO .
- ³¹P NMR : Distinguishes between free and metal-bound DppfO₂ ligands.
- Cyclic voltammetry : Probes redox behavior, such as the ferrocene/ferrocenium couple (~0 V vs. Ag/AgCl) and ligand-influenced shifts in metal-centered redox potentials .
Q. What safety protocols are essential when handling DppfO₂ in laboratory settings?
- Guidelines :
- Personal protective equipment (PPE) : Wear nitrile gloves, lab coats, and safety goggles to prevent skin/eye contact.
- Ventilation : Use fume hoods to avoid inhalation of dust/aerosols.
- Storage : Keep under inert atmosphere (argon/nitrogen) in airtight containers to prevent oxidation or moisture uptake .
- Spill management : Avoid dry sweeping; use wet methods or HEPA vacuums to contain particulates .
Advanced Research Questions
Q. How can density functional theory (DFT) calculations elucidate the electronic structure and catalytic activity of DppfO₂-metal complexes?
- Approach : DFT studies optimize geometries and compute frontier molecular orbitals (HOMO/LUMO) to predict redox-active sites. For instance, DFT modeling of [Fe₂(CO)₅(κ¹-DppfO)(µ-benzenedithiolate)] revealed ligand-to-metal charge transfer (LMCT) pathways critical for proton reduction, mimicking hydrogenase enzymes . Parameters like Mayer bond orders and spin densities help rationalize experimental observations, such as unexpected regioselectivity in catalytic cycles .
Q. What strategies resolve contradictions in catalytic performance data for DppfO₂-based systems (e.g., turnover frequency variations)?
- Analysis :
- Control experiments : Isolate variables like solvent polarity (e.g., THF vs. DMF), temperature, and counterion effects.
- In-situ spectroscopy : Use IR or UV-vis to monitor intermediate species during catalysis.
- Kinetic isotope effects (KIE) : Probe rate-determining steps (e.g., H-H bond formation vs. electron transfer) .
- Cross-referencing : Compare with structurally analogous ligands (e.g., Dppf vs. DppfO₂) to identify steric/electronic contributions to activity .
Q. How does DppfO₂ enhance the stability and selectivity of palladium catalysts in cross-coupling reactions compared to non-oxidized ligands?
- Mechanistic insights : DppfO₂’s stronger σ-donor and weaker π-acceptor properties stabilize Pd(0) intermediates, reducing catalyst deactivation via aggregation. The rigid ferrocene backbone enforces cis coordination geometry, improving selectivity in Suzuki-Miyaura couplings. Comparative studies with Pd(Dppf)Cl₂ show higher yields (85–92% vs. 70–78%) and tolerance for electron-deficient aryl halides .
Q. What experimental and computational approaches optimize DppfO₂’s ligand design for targeted applications (e.g., CO₂ reduction or asymmetric catalysis)?
- Design framework :
- Steric tuning : Introduce substituents (e.g., electron-withdrawing groups on phenyl rings) to modulate metal-ligand bond strength.
- Chiral derivatization : Attach chiral auxiliaries (e.g., binaphthyl groups) to enable enantioselective transformations.
- High-throughput screening : Test ligand libraries under varied conditions to identify structure-activity trends .
Properties
IUPAC Name |
[cyclopenta-1,3-dien-1-yl(phenyl)phosphoryl]benzene;iron(2+) | |
---|---|---|
Source | PubChem | |
URL | https://pubchem.ncbi.nlm.nih.gov | |
Description | Data deposited in or computed by PubChem | |
InChI |
InChI=1S/2C17H14OP.Fe/c2*18-19(17-13-7-8-14-17,15-9-3-1-4-10-15)16-11-5-2-6-12-16;/h2*1-14H;/q2*-1;+2 | |
Source | PubChem | |
URL | https://pubchem.ncbi.nlm.nih.gov | |
Description | Data deposited in or computed by PubChem | |
InChI Key |
CSCOLJZENYWZRC-UHFFFAOYSA-N | |
Source | PubChem | |
URL | https://pubchem.ncbi.nlm.nih.gov | |
Description | Data deposited in or computed by PubChem | |
Canonical SMILES |
[CH-]1C=CC=C1P(=O)(C2=CC=CC=C2)C3=CC=CC=C3.[CH-]1C=CC=C1P(=O)(C2=CC=CC=C2)C3=CC=CC=C3.[Fe+2] | |
Source | PubChem | |
URL | https://pubchem.ncbi.nlm.nih.gov | |
Description | Data deposited in or computed by PubChem | |
Molecular Formula |
C34H28FeO2P2 | |
Source | PubChem | |
URL | https://pubchem.ncbi.nlm.nih.gov | |
Description | Data deposited in or computed by PubChem | |
Molecular Weight |
586.4 g/mol | |
Source | PubChem | |
URL | https://pubchem.ncbi.nlm.nih.gov | |
Description | Data deposited in or computed by PubChem | |
Retrosynthesis Analysis
AI-Powered Synthesis Planning: Our tool employs the Template_relevance Pistachio, Template_relevance Bkms_metabolic, Template_relevance Pistachio_ringbreaker, Template_relevance Reaxys, Template_relevance Reaxys_biocatalysis model, leveraging a vast database of chemical reactions to predict feasible synthetic routes.
One-Step Synthesis Focus: Specifically designed for one-step synthesis, it provides concise and direct routes for your target compounds, streamlining the synthesis process.
Accurate Predictions: Utilizing the extensive PISTACHIO, BKMS_METABOLIC, PISTACHIO_RINGBREAKER, REAXYS, REAXYS_BIOCATALYSIS database, our tool offers high-accuracy predictions, reflecting the latest in chemical research and data.
Strategy Settings
Precursor scoring | Relevance Heuristic |
---|---|
Min. plausibility | 0.01 |
Model | Template_relevance |
Template Set | Pistachio/Bkms_metabolic/Pistachio_ringbreaker/Reaxys/Reaxys_biocatalysis |
Top-N result to add to graph | 6 |
Feasible Synthetic Routes
Disclaimer and Information on In-Vitro Research Products
Please be aware that all articles and product information presented on BenchChem are intended solely for informational purposes. The products available for purchase on BenchChem are specifically designed for in-vitro studies, which are conducted outside of living organisms. In-vitro studies, derived from the Latin term "in glass," involve experiments performed in controlled laboratory settings using cells or tissues. It is important to note that these products are not categorized as medicines or drugs, and they have not received approval from the FDA for the prevention, treatment, or cure of any medical condition, ailment, or disease. We must emphasize that any form of bodily introduction of these products into humans or animals is strictly prohibited by law. It is essential to adhere to these guidelines to ensure compliance with legal and ethical standards in research and experimentation.