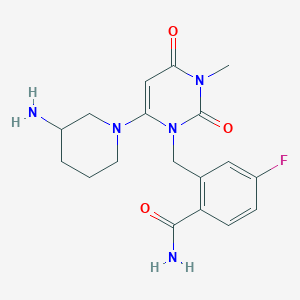
Trelagliptin Impurity X
Overview
Description
Trelagliptin Impurity X is a byproduct or degradation product associated with the synthesis and stability of Trelagliptin, a dipeptidyl peptidase-4 (DPP-4) inhibitor used in the treatment of type 2 diabetes. Impurities in pharmaceutical compounds are critical to identify and control as they can affect the efficacy and safety of the drug.
Mechanism of Action
Target of Action
Mode of Action
Biochemical Pathways
Trelagliptin affects the AMPK pathway . It has been observed to significantly activate the AMPK pathway in oxygen-glucose deprivation/reperfusion (OGD/R)-challenged human aortic valvular endothelial cells (HAVECs) . This activation leads to the amelioration of OGD/R-induced mitochondrial disturbance and metabolic changes .
Pharmacokinetics
The pharmacokinetic properties of Trelagliptin are characterized by its slow-binding inhibition of DPP-4 . The half-life for dissociation (t1/2) is approximately 30 minutes . .
Result of Action
Action Environment
The action of Trelagliptin can be influenced by environmental factors such as the presence of oxygen-glucose deprivation/reperfusion (OGD/R) conditions . In such conditions, Trelagliptin has been observed to exert a protective effect against mitochondrial dysfunction and metabolic disturbance . .
Biochemical Analysis
Biochemical Properties
Trelagliptin Impurity X plays a role in biochemical reactions primarily through its interactions with enzymes, proteins, and other biomolecules. It has been observed to interact with dipeptidyl peptidase-4 (DPP-4), similar to Trelagliptin itself. The nature of these interactions involves reversible, competitive, and slow-binding inhibition of DPP-4 . This interaction is significant because DPP-4 is responsible for the inactivation of incretin hormones, which play a crucial role in regulating blood glucose levels.
Cellular Effects
The effects of this compound on various types of cells and cellular processes are noteworthy. It influences cell function by modulating cell signaling pathways, gene expression, and cellular metabolism. Studies have shown that this compound can affect beta-cell function in patients with type 2 diabetes, leading to changes in insulin secretion and resistance . Additionally, it has been observed to impact body composition by reducing body fat mass without affecting skeletal muscle mass .
Molecular Mechanism
At the molecular level, this compound exerts its effects through specific binding interactions with biomolecules. It inhibits dipeptidyl peptidase-4 (DPP-4) by forming a non-covalent interaction, which results in the stabilization of incretin hormones and enhanced insulin secretion . This inhibition mechanism is crucial for its role in controlling blood glucose levels in diabetic patients.
Temporal Effects in Laboratory Settings
The temporal effects of this compound in laboratory settings have been studied to understand its stability, degradation, and long-term effects on cellular function. Over time, this compound has shown consistent inhibition of DPP-4 activity, which contributes to its sustained efficacy
Dosage Effects in Animal Models
The effects of this compound at different dosages have been studied in animal models to determine its threshold and toxic effects. It has been observed that higher doses of this compound can lead to adverse effects, including potential toxicity . Understanding the dosage effects is essential for determining safe and effective therapeutic levels.
Metabolic Pathways
This compound is involved in various metabolic pathways, interacting with enzymes and cofactors that influence its metabolic flux and metabolite levels. The inhibition of DPP-4 by this compound affects the metabolism of incretin hormones, leading to increased insulin secretion and improved glucose regulation .
Transport and Distribution
The transport and distribution of this compound within cells and tissues involve specific transporters and binding proteins. These interactions affect its localization and accumulation, which are critical for its pharmacological activity . Understanding these transport mechanisms is essential for optimizing its therapeutic use.
Subcellular Localization
The subcellular localization of this compound plays a significant role in its activity and function. It is directed to specific compartments or organelles through targeting signals and post-translational modifications . This localization is crucial for its interaction with DPP-4 and subsequent inhibition of the enzyme.
Preparation Methods
The preparation of Trelagliptin Impurity X involves several synthetic routes and reaction conditions. One method includes dissolving the compound in ethanol, adding sodium bicarbonate, and reacting at elevated temperatures . The process is designed to be efficient, with easily available raw materials, strong operability, convenient purification, and simple post-treatment steps . Industrial production methods focus on optimizing these steps to produce large quantities of the impurity for further analysis and quality control.
Chemical Reactions Analysis
Trelagliptin Impurity X undergoes various chemical reactions, including oxidation, reduction, and substitution. Common reagents used in these reactions include oxidizing agents, reducing agents, and nucleophiles. The major products formed from these reactions depend on the specific conditions and reagents used. For example, oxidation reactions may produce different oxidized forms of the impurity, while substitution reactions can lead to the formation of various substituted derivatives .
Scientific Research Applications
Trelagliptin Impurity X has several scientific research applications. It is used in pharmaceutical research for product development, quality control, method validation, and stability studies . Additionally, it is useful in the identification of unknown impurities and the assessment of genotoxic potential . In chemistry, it helps in understanding the degradation pathways and stability of Trelagliptin. In biology and medicine, it aids in evaluating the safety and efficacy of the drug by studying the effects of impurities.
Comparison with Similar Compounds
Trelagliptin Impurity X can be compared with other impurities and degradation products of similar DPP-4 inhibitors, such as Alogliptin, Saxagliptin, and Sitagliptin . These compounds share a similar mechanism of action but may differ in their impurity profiles and stability. This compound is unique in its specific chemical structure and the conditions under which it is formed. Understanding these differences is crucial for ensuring the safety and efficacy of the parent drug.
Conclusion
This compound is an important compound in the study of pharmaceutical impurities. Its preparation, chemical reactions, and scientific research applications provide valuable insights into the stability and safety of Trelagliptin. Further research is needed to fully understand its mechanism of action and to compare it with similar compounds.
Biological Activity
Trelagliptin, a dipeptidyl peptidase-4 (DPP-4) inhibitor, is primarily used for the treatment of type 2 diabetes mellitus. Trelagliptin Impurity X refers to an impurity associated with the drug, which may have implications for its biological activity and therapeutic efficacy. This article explores the biological activity of this compound, focusing on its mechanism of action, pharmacological effects, and relevant research findings.
Trelagliptin acts by inhibiting the DPP-4 enzyme, which is responsible for the degradation of incretin hormones such as glucagon-like peptide-1 (GLP-1). By inhibiting DPP-4, trelagliptin increases the levels of GLP-1, leading to enhanced insulin secretion and reduced glucagon levels in response to meals. This mechanism contributes to improved glycemic control in patients with type 2 diabetes .
Inhibition of DPP-4 Activity
Research has demonstrated that this compound exhibits a concentration-dependent inhibition of DPP-4 activity. The inhibitory effect was assessed using recombinant human DPP-4 and various animal models. The 50% inhibitory concentration (IC50) values for trelagliptin were found to be as follows:
Species | IC50 (nmol/L) |
---|---|
Human | 5.4 [95% CI: 5.2-5.7] |
Dog | 6.2 [95% CI: 6.0-6.4] |
Rat | 9.7 [95% CI: 8.0-11.8] |
The mode of inhibition was characterized as competitive and reversible, with a dissociation half-life of approximately 30 minutes .
Pharmacokinetics and Efficacy
Trelagliptin is administered once weekly, demonstrating favorable pharmacokinetic properties that enhance patient compliance. Studies have indicated that the drug reaches peak plasma concentrations within 1 to 1.5 hours post-administration, with a half-life that supports its once-weekly dosing regimen .
In clinical trials, trelagliptin has shown significant efficacy in reducing HbA1c levels compared to placebo and other antidiabetic agents. For instance, a multicenter study reported an overall incidence of treatment-emergent adverse events (TEAEs) at 87.9% in patients receiving trelagliptin as an add-on therapy to insulin .
Case Studies and Clinical Findings
Several studies have investigated the effects of trelagliptin in diverse patient populations:
- Combination Therapy with Insulin : A study assessed the efficacy and safety of trelagliptin combined with insulin in patients who were unable to achieve glycemic control with insulin alone. The results indicated that trelagliptin effectively lowered blood glucose levels without increasing the risk of severe hypoglycemia .
- Long-term Efficacy : Another long-term study evaluated the effects of switching to trelagliptin from other DPP-4 inhibitors or sulfonylureas. Patients experienced sustained reductions in HbA1c over a follow-up period, highlighting the drug's effectiveness as a treatment option for type 2 diabetes .
- Adverse Event Profile : While generally well-tolerated, some adverse events were reported, including gastrointestinal symptoms and mild skin reactions. The overall safety profile suggests that trelagliptin is a viable option for many patients with type 2 diabetes .
Properties
IUPAC Name |
2-[[6-(3-aminopiperidin-1-yl)-3-methyl-2,4-dioxopyrimidin-1-yl]methyl]-4-fluorobenzamide | |
---|---|---|
Source | PubChem | |
URL | https://pubchem.ncbi.nlm.nih.gov | |
Description | Data deposited in or computed by PubChem | |
InChI |
InChI=1S/C18H22FN5O3/c1-22-16(25)8-15(23-6-2-3-13(20)10-23)24(18(22)27)9-11-7-12(19)4-5-14(11)17(21)26/h4-5,7-8,13H,2-3,6,9-10,20H2,1H3,(H2,21,26) | |
Source | PubChem | |
URL | https://pubchem.ncbi.nlm.nih.gov | |
Description | Data deposited in or computed by PubChem | |
InChI Key |
AKNPUJHLPMLIQG-UHFFFAOYSA-N | |
Source | PubChem | |
URL | https://pubchem.ncbi.nlm.nih.gov | |
Description | Data deposited in or computed by PubChem | |
Canonical SMILES |
CN1C(=O)C=C(N(C1=O)CC2=C(C=CC(=C2)F)C(=O)N)N3CCCC(C3)N | |
Source | PubChem | |
URL | https://pubchem.ncbi.nlm.nih.gov | |
Description | Data deposited in or computed by PubChem | |
Molecular Formula |
C18H22FN5O3 | |
Source | PubChem | |
URL | https://pubchem.ncbi.nlm.nih.gov | |
Description | Data deposited in or computed by PubChem | |
Molecular Weight |
375.4 g/mol | |
Source | PubChem | |
URL | https://pubchem.ncbi.nlm.nih.gov | |
Description | Data deposited in or computed by PubChem | |
Retrosynthesis Analysis
AI-Powered Synthesis Planning: Our tool employs the Template_relevance Pistachio, Template_relevance Bkms_metabolic, Template_relevance Pistachio_ringbreaker, Template_relevance Reaxys, Template_relevance Reaxys_biocatalysis model, leveraging a vast database of chemical reactions to predict feasible synthetic routes.
One-Step Synthesis Focus: Specifically designed for one-step synthesis, it provides concise and direct routes for your target compounds, streamlining the synthesis process.
Accurate Predictions: Utilizing the extensive PISTACHIO, BKMS_METABOLIC, PISTACHIO_RINGBREAKER, REAXYS, REAXYS_BIOCATALYSIS database, our tool offers high-accuracy predictions, reflecting the latest in chemical research and data.
Strategy Settings
Precursor scoring | Relevance Heuristic |
---|---|
Min. plausibility | 0.01 |
Model | Template_relevance |
Template Set | Pistachio/Bkms_metabolic/Pistachio_ringbreaker/Reaxys/Reaxys_biocatalysis |
Top-N result to add to graph | 6 |
Feasible Synthetic Routes
Disclaimer and Information on In-Vitro Research Products
Please be aware that all articles and product information presented on BenchChem are intended solely for informational purposes. The products available for purchase on BenchChem are specifically designed for in-vitro studies, which are conducted outside of living organisms. In-vitro studies, derived from the Latin term "in glass," involve experiments performed in controlled laboratory settings using cells or tissues. It is important to note that these products are not categorized as medicines or drugs, and they have not received approval from the FDA for the prevention, treatment, or cure of any medical condition, ailment, or disease. We must emphasize that any form of bodily introduction of these products into humans or animals is strictly prohibited by law. It is essential to adhere to these guidelines to ensure compliance with legal and ethical standards in research and experimentation.