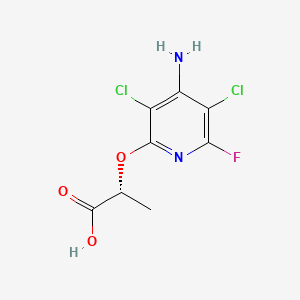
Fluchloraminopyr
- Click on QUICK INQUIRY to receive a quote from our team of experts.
- With the quality product at a COMPETITIVE price, you can focus more on your research.
Overview
Description
Fluchloraminopyr is a novel broad-spectrum herbicide developed by KingAgroot, an agricultural biotechnology company in China. It is a pyridyloxycarboxylic acid herbicide with the chemical formula C₈H₇Cl₂FN₂O₃ and a molecular mass of 269.06 g/mol . This compound is known for its effectiveness in controlling a wide range of weeds, including those resistant to glyphosate .
Preparation Methods
Fluchloraminopyr is synthesized through a series of chemical reactions. The industrial production method involves the use of specific reaction conditions to ensure high yield and purity of the final product .
Chemical Reactions Analysis
Fluchloraminopyr undergoes various chemical reactions, including oxidation, reduction, and substitution. Common reagents used in these reactions include oxidizing agents, reducing agents, and nucleophiles. The major products formed from these reactions depend on the specific conditions and reagents used . For example, oxidation of this compound can lead to the formation of its corresponding oxoacid .
Scientific Research Applications
Herbicidal Properties
Fluchloraminopyr exhibits excellent herbicidal activity against a range of grass weeds and broadleaf species. It has been tested on various crops including:
- Cereal Crops : Wheat, corn, and rice.
- Cash Crops : Soybean, cotton, oil sunflower, and sugarcane.
- Vegetables and Fruits : Potatoes and various fruit trees.
The compound shows significant selectivity for established grass lawns such as Zoysia, Bermudagrass, tall fescue, Bluegrass, ryegrass, and seaside paspalum. This selectivity allows for effective weed control without harming desirable turf grasses .
Environmental Impact and Degradation
Research on the degradation of this compound in soil indicates that it undergoes various transformation processes. A study conducted under aerobic and anaerobic conditions revealed:
- Dissipation Time : The compound's Dissipation Time 50 (DT50) values ranged from 0.107 to 4.76 days across different soil types.
- Transformation Products : Eight transformation products were identified through advanced techniques like Ultra-High Performance Liquid Chromatography coupled with Quadrupole Time-of-Flight Mass Spectrometry (UHPLC-QTOF/MS). The primary degradation pathways included oxidation, dechlorination, hydroxylation, and acetylation.
Toxicological assessments suggested that the acute and chronic toxicity of these transformation products to aquatic organisms was significantly lower than that of the parent compound . This finding is crucial for ecological risk evaluations and supports the judicious application of this compound in agricultural settings.
Case Study 1: Efficacy in Rice Cultivation
In trials conducted in rice paddies, this compound demonstrated superior efficacy in controlling common weeds while ensuring high crop safety. The selectivity for rice was particularly noted, making it a valuable tool for rice farmers looking to manage weed populations without compromising yield .
Case Study 2: Comparative Analysis with Other Herbicides
A comparative study evaluated this compound against traditional herbicides like glyphosate. Results indicated that this compound provided comparable or superior weed control with a significantly reduced risk of environmental contamination due to its rapid degradation profile .
Table: Comparison of Herbicidal Efficacy
Crop Type | Weed Control Efficacy | Crop Safety | Environmental Impact |
---|---|---|---|
Rice | High | High | Low |
Wheat | Moderate | High | Low |
Soybean | High | Moderate | Moderate |
Turf Grasses | High | Very High | Low |
Mechanism of Action
The mechanism of action of fluchloraminopyr involves its absorption by plants, where it interferes with nucleic acid and protein synthesis. This leads to excessive cell division and elongation, resulting in abnormal growth and eventual death of the plant . The molecular targets and pathways involved in this process are still under investigation, but it is believed to be similar to that of other synthetic auxin herbicides .
Comparison with Similar Compounds
Fluchloraminopyr is unique among herbicides due to its broad-spectrum activity and effectiveness against glyphosate-resistant weeds. Similar compounds include fluroxypyr, cypyrafluone, bipyrazone, fenpyrazone, and tripyrasulfone . Compared to these compounds, this compound has a longer residual activity and better performance under low-temperature conditions .
Biological Activity
Fluchloraminopyr is a novel herbicide that has garnered attention for its potential applications in agricultural systems. This article explores its biological activity, degradation processes, ecological risks, and relevant case studies.
This compound belongs to the pyridine herbicide class and exhibits selective herbicidal activity against a variety of grass and broadleaf weeds. Its chemical structure allows it to inhibit specific biochemical pathways in target plants, disrupting their growth and development. The primary mechanism involves interference with the synthesis of essential amino acids, ultimately leading to plant death.
Degradation in Soil
Research indicates that the degradation of this compound in soil occurs through several transformation pathways, including oxidation, dechlorination, hydroxylation, and acetylation. A study evaluated the dissipation of this compound under both aerobic and anaerobic conditions across four representative soils. The Dissipation Time 50 (DT50) values ranged from 0.107 to 4.76 days, suggesting rapid degradation under certain conditions .
Transformation Products
The study identified eight transformation products (TPs) using Ultra-High Performance Liquid Chromatography coupled with Quadrupole Time-of-Flight Mass Spectrometry (UHPLC-QTOF/MS). Notably, two TPs, M267 and M221, exhibited higher acute oral toxicity to terrestrial organisms compared to this compound itself .
Transformation Product | Acute Oral Toxicity | Notes |
---|---|---|
This compound | Lower | Parent compound |
M267 | Higher | More toxic than parent |
M221 | Higher | More toxic than parent |
Ecological Risk Assessment
The ecological risk assessment of this compound indicates that while the parent compound poses significant risks to aquatic life, its transformation products may present lower toxicity levels. Predictions from toxicological software indicated that both acute and chronic toxicity of TPs to aquatic organisms was significantly lower than that of this compound .
Case Studies
Several case studies have documented the impacts of this compound in agricultural settings:
- Field Trials : Field trials conducted on various crops such as wheat, corn, and soybeans demonstrated excellent selectivity and efficacy against key grass weeds while maintaining safety for the crops .
- Environmental Impact Studies : Studies evaluating the environmental fate of this compound highlighted its rapid degradation in soil, which is crucial for minimizing long-term ecological impacts .
- Toxicity Assessments : Research assessing the toxicity of this compound's transformation products found that while some metabolites are more toxic than the parent compound, their overall impact on non-target species remains low .
Properties
CAS No. |
2445980-81-2 |
---|---|
Molecular Formula |
C8H7Cl2FN2O3 |
Molecular Weight |
269.05 g/mol |
IUPAC Name |
(2R)-2-(4-amino-3,5-dichloro-6-fluoropyridin-2-yl)oxypropanoic acid |
InChI |
InChI=1S/C8H7Cl2FN2O3/c1-2(8(14)15)16-7-4(10)5(12)3(9)6(11)13-7/h2H,1H3,(H2,12,13)(H,14,15)/t2-/m1/s1 |
InChI Key |
LEIWLFHGOGNSAD-UWTATZPHSA-N |
Isomeric SMILES |
C[C@H](C(=O)O)OC1=NC(=C(C(=C1Cl)N)Cl)F |
Canonical SMILES |
CC(C(=O)O)OC1=NC(=C(C(=C1Cl)N)Cl)F |
Origin of Product |
United States |
Disclaimer and Information on In-Vitro Research Products
Please be aware that all articles and product information presented on BenchChem are intended solely for informational purposes. The products available for purchase on BenchChem are specifically designed for in-vitro studies, which are conducted outside of living organisms. In-vitro studies, derived from the Latin term "in glass," involve experiments performed in controlled laboratory settings using cells or tissues. It is important to note that these products are not categorized as medicines or drugs, and they have not received approval from the FDA for the prevention, treatment, or cure of any medical condition, ailment, or disease. We must emphasize that any form of bodily introduction of these products into humans or animals is strictly prohibited by law. It is essential to adhere to these guidelines to ensure compliance with legal and ethical standards in research and experimentation.