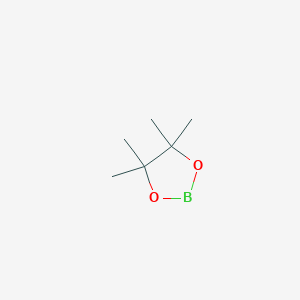
4,4,5,5-Tetramethyl-1,3,2-dioxaborolane
Overview
Description
Mechanism of Action
Target of Action
Pinacolborane, also known as 1,3,2-Dioxaborolane, 4,4,5,5-tetramethyl-, primarily targets alkenes , alkynes , aldehydes , ketones , and carboxylic acids . These compounds are the primary substrates that interact with Pinacolborane in various chemical reactions.
Mode of Action
Pinacolborane interacts with its targets through a process called hydroboration . In the presence of catalysts, Pinacolborane hydroborates alkenes and, less rapidly, alkynes . It also affects catalyst-free hydroboration of aldehydes, ketones, and carboxylic acids . The active hydride source in these reactions is the trialkoxyborohydride .
Biochemical Pathways
The primary biochemical pathway involved in the action of Pinacolborane is the reductive amination of carbonyl compounds . This process involves the reduction of ketones with Pinacolborane, catalyzed by NaOt-Bu at ambient temperature . The reaction is high yielding and general, providing complete conversion of aryl and dialkyl ketones .
Result of Action
The result of Pinacolborane’s action is the formation of boron-containing compounds . For example, dehydrogenation of Pinacolborane affords dipinacolatodiborane (B2pin2) . Moreover, Pinacolborane is used in borylation, a form of C-H activation .
Action Environment
The action of Pinacolborane can be influenced by various environmental factors. For instance, the reaction temperature and the weight ratio of reactants can affect the outcome of the reaction . Additionally, the presence of catalysts can significantly enhance the efficiency of the hydroboration process .
Preparation Methods
Synthetic Routes and Reaction Conditions
4,4,5,5-Tetramethyl-1,3,2-dioxaborolane can be synthesized through the reaction of pinacol (2,3-dimethyl-2,3-butanediol) with borane (BH₃) in tetrahydrofuran (THF) solvent. The reaction typically proceeds at room temperature and yields pinacolborane as a colorless liquid .
Industrial Production Methods
Industrial production of pinacolborane involves similar synthetic routes but on a larger scale. The reaction conditions are optimized to ensure high yield and purity of the product. The process may involve continuous flow reactors to maintain consistent reaction conditions and improve efficiency .
Chemical Reactions Analysis
Types of Reactions
4,4,5,5-Tetramethyl-1,3,2-dioxaborolane undergoes various types of chemical reactions, including:
Hydroboration: This compound reacts with alkenes and alkynes to form organoboranes.
Reduction: It reduces ketones and aldehydes to their corresponding alcohols.
Borylation: This compound is used in borylation reactions to introduce boron atoms into organic molecules.
Common Reagents and Conditions
Hydroboration: Catalysts such as nickel or cobalt are often used to facilitate the hydroboration of alkenes and alkynes.
Reduction: Sodium tert-butoxide (NaOtBu) is commonly used as a catalyst for the reduction of ketones and aldehydes.
Borylation: Transition metal catalysts like palladium are used in borylation reactions.
Major Products Formed
Hydroboration: Organoboranes
Reduction: Alcohols
Borylation: Boronic esters
Scientific Research Applications
4,4,5,5-Tetramethyl-1,3,2-dioxaborolane has a wide range of applications in scientific research:
Comparison with Similar Compounds
Similar Compounds
Catecholborane: Another borane compound used in hydroboration reactions.
Dicyclohexylborane: Used in hydroboration of alkynes.
Uniqueness of 4,4,5,5-Tetramethyl-1,3,2-dioxaborolane
This compound is unique due to its high reactivity and selectivity in hydroboration reactions. It is also versatile, being used in both catalyst-free and catalyst-assisted reactions . Additionally, pinacolborane’s ability to form stable boronic esters makes it valuable in organic synthesis .
Biological Activity
4,4,5,5-Tetramethyl-1,3,2-dioxaborolane is a boron-containing compound with significant potential in organic synthesis and medicinal chemistry. Its unique structure allows it to participate in various chemical reactions while exhibiting notable biological activities. This article explores the biological activity of this compound, including its mechanisms of action, potential therapeutic applications, and relevant case studies.
Chemical Structure and Properties
The molecular formula of this compound is with a molecular weight of approximately 127.98 g/mol. The compound features a dioxaborolane ring that stabilizes the boron atom, enhancing its reactivity in organic transformations. The presence of the tetramethyl substituents contributes to its steric properties and solubility.
Research indicates that this compound exhibits significant biological activity primarily through its interaction with cytochrome P450 enzymes. Specifically:
- Inhibition of Cytochrome P450 Enzymes : This compound has been shown to inhibit CYP1A2 and CYP2D6 enzymes which are crucial for drug metabolism. This inhibition can lead to altered pharmacokinetics for drugs metabolized by these pathways.
Pharmacological Applications
The potential applications of this compound in pharmacology include:
- Drug Development : Its ability to inhibit specific cytochrome P450 enzymes suggests it could be used to enhance the efficacy of certain drugs by preventing their rapid metabolism.
- Synthetic Intermediate : It serves as a valuable intermediate in the synthesis of biologically active compounds due to its reactivity in cross-coupling reactions such as Suzuki-Miyaura coupling.
Study 1: Interaction with Drug Metabolism
A study published in the Journal of Medicinal Chemistry examined the effects of this compound on drug metabolism. The results indicated that co-administration with substrates for CYP1A2 and CYP2D6 led to significant increases in plasma concentrations of those drugs. This finding highlights the compound's potential as a pharmacological agent that can modulate drug interactions.
Study 2: Synthesis and Biological Evaluation
In another study focusing on the synthesis of derivatives of this compound for biological evaluation:
- Various derivatives were synthesized and tested against different cancer cell lines.
- Some derivatives exhibited promising cytotoxicity and were further investigated for their mechanisms of action involving apoptosis induction .
Comparative Analysis
The following table summarizes structural similarities and biological activities among related compounds:
Compound Name | CAS Number | Biological Activity | Unique Features |
---|---|---|---|
2-(Benzofuran-7-yl)-4,4,5,5-tetramethyl-1,3,2-dioxaborolane | 1192755-14-8 | Inhibitor of CYP enzymes | Different substitution pattern |
2-Methoxy-5-(4,4,5,5-tetramethyl-1,3,2-dioxaborolan-2-yl)benzenemethanol | 1009303-77-8 | Moderate cytotoxicity | Contains methoxy group |
2-(Benzofuran-5-yl)-4,4,5,5-tetramethyl-1,3,2-dioxaborolane | 519054-55-8 | Potential anti-cancer activity | Lacks chlorine substituent |
Properties
InChI |
InChI=1S/C6H12BO2/c1-5(2)6(3,4)9-7-8-5/h1-4H3 | |
---|---|---|
Source | PubChem | |
URL | https://pubchem.ncbi.nlm.nih.gov | |
Description | Data deposited in or computed by PubChem | |
InChI Key |
LZPWAYBEOJRFAX-UHFFFAOYSA-N | |
Source | PubChem | |
URL | https://pubchem.ncbi.nlm.nih.gov | |
Description | Data deposited in or computed by PubChem | |
Canonical SMILES |
[B]1OC(C(O1)(C)C)(C)C | |
Source | PubChem | |
URL | https://pubchem.ncbi.nlm.nih.gov | |
Description | Data deposited in or computed by PubChem | |
Molecular Formula |
C6H12BO2 | |
Source | PubChem | |
URL | https://pubchem.ncbi.nlm.nih.gov | |
Description | Data deposited in or computed by PubChem | |
DSSTOX Substance ID |
DTXSID30422852 | |
Record name | Pinacolborane | |
Source | EPA DSSTox | |
URL | https://comptox.epa.gov/dashboard/DTXSID30422852 | |
Description | DSSTox provides a high quality public chemistry resource for supporting improved predictive toxicology. | |
Molecular Weight |
126.97 g/mol | |
Source | PubChem | |
URL | https://pubchem.ncbi.nlm.nih.gov | |
Description | Data deposited in or computed by PubChem | |
CAS No. |
25015-63-8 | |
Record name | 1,3,2-Dioxaborolane, 4,4,5,5-tetramethyl- | |
Source | ChemIDplus | |
URL | https://pubchem.ncbi.nlm.nih.gov/substance/?source=chemidplus&sourceid=0025015638 | |
Description | ChemIDplus is a free, web search system that provides access to the structure and nomenclature authority files used for the identification of chemical substances cited in National Library of Medicine (NLM) databases, including the TOXNET system. | |
Record name | Pinacolborane | |
Source | EPA DSSTox | |
URL | https://comptox.epa.gov/dashboard/DTXSID30422852 | |
Description | DSSTox provides a high quality public chemistry resource for supporting improved predictive toxicology. | |
Record name | 4,4,5,5-Tetramethyl-1,3,2-dioxaborolane | |
Source | European Chemicals Agency (ECHA) | |
URL | https://echa.europa.eu/substance-information/-/substanceinfo/100.118.700 | |
Description | The European Chemicals Agency (ECHA) is an agency of the European Union which is the driving force among regulatory authorities in implementing the EU's groundbreaking chemicals legislation for the benefit of human health and the environment as well as for innovation and competitiveness. | |
Explanation | Use of the information, documents and data from the ECHA website is subject to the terms and conditions of this Legal Notice, and subject to other binding limitations provided for under applicable law, the information, documents and data made available on the ECHA website may be reproduced, distributed and/or used, totally or in part, for non-commercial purposes provided that ECHA is acknowledged as the source: "Source: European Chemicals Agency, http://echa.europa.eu/". Such acknowledgement must be included in each copy of the material. ECHA permits and encourages organisations and individuals to create links to the ECHA website under the following cumulative conditions: Links can only be made to webpages that provide a link to the Legal Notice page. | |
Retrosynthesis Analysis
AI-Powered Synthesis Planning: Our tool employs the Template_relevance Pistachio, Template_relevance Bkms_metabolic, Template_relevance Pistachio_ringbreaker, Template_relevance Reaxys, Template_relevance Reaxys_biocatalysis model, leveraging a vast database of chemical reactions to predict feasible synthetic routes.
One-Step Synthesis Focus: Specifically designed for one-step synthesis, it provides concise and direct routes for your target compounds, streamlining the synthesis process.
Accurate Predictions: Utilizing the extensive PISTACHIO, BKMS_METABOLIC, PISTACHIO_RINGBREAKER, REAXYS, REAXYS_BIOCATALYSIS database, our tool offers high-accuracy predictions, reflecting the latest in chemical research and data.
Strategy Settings
Precursor scoring | Relevance Heuristic |
---|---|
Min. plausibility | 0.01 |
Model | Template_relevance |
Template Set | Pistachio/Bkms_metabolic/Pistachio_ringbreaker/Reaxys/Reaxys_biocatalysis |
Top-N result to add to graph | 6 |
Feasible Synthetic Routes
Disclaimer and Information on In-Vitro Research Products
Please be aware that all articles and product information presented on BenchChem are intended solely for informational purposes. The products available for purchase on BenchChem are specifically designed for in-vitro studies, which are conducted outside of living organisms. In-vitro studies, derived from the Latin term "in glass," involve experiments performed in controlled laboratory settings using cells or tissues. It is important to note that these products are not categorized as medicines or drugs, and they have not received approval from the FDA for the prevention, treatment, or cure of any medical condition, ailment, or disease. We must emphasize that any form of bodily introduction of these products into humans or animals is strictly prohibited by law. It is essential to adhere to these guidelines to ensure compliance with legal and ethical standards in research and experimentation.