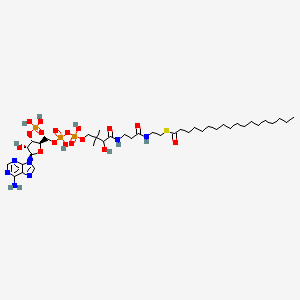
Coenzyme A,S-octadecanoate
- Click on QUICK INQUIRY to receive a quote from our team of experts.
- With the quality product at a COMPETITIVE price, you can focus more on your research.
Overview
Description
Coenzyme A,S-octadecanoate is a derivative of coenzyme A, which is a crucial cofactor in various biochemical processes. Coenzyme A plays a significant role in the synthesis and oxidation of fatty acids, as well as the oxidation of pyruvate in the citric acid cycle. This compound specifically involves the esterification of coenzyme A with octadecanoic acid (stearic acid), resulting in a compound that is essential for various metabolic pathways .
Preparation Methods
Synthetic Routes and Reaction Conditions
The synthesis of Coenzyme A,S-octadecanoate typically involves a chemo-enzymatic approach. One common method includes the activation of octadecanoic acid to its corresponding acyl-CoA derivative using acyl-CoA synthetase. This reaction requires adenosine triphosphate (ATP) and coenzyme A as substrates, and it is catalyzed by the enzyme acyl-CoA synthetase under physiological conditions .
Industrial Production Methods
Industrial production of this compound often involves biotechnological processes using microbial fermentation. Microorganisms such as Escherichia coli can be genetically engineered to overproduce the necessary enzymes for the biosynthesis of coenzyme A derivatives. The fermentation process is optimized to achieve high yields of the desired product, which is then purified using chromatographic techniques .
Chemical Reactions Analysis
Types of Reactions
Coenzyme A,S-octadecanoate undergoes various biochemical reactions, including:
Oxidation: Involved in the beta-oxidation pathway of fatty acids, where it is converted to acetyl-CoA.
Reduction: Participates in the reduction of fatty acids during biosynthesis.
Substitution: Acts as a substrate in transesterification reactions, where the octadecanoate group is transferred to other molecules.
Common Reagents and Conditions
Oxidation: Requires enzymes such as acyl-CoA dehydrogenase, enoyl-CoA hydratase, and beta-hydroxyacyl-CoA dehydrogenase.
Reduction: Involves enzymes like fatty acid synthase and reductases.
Substitution: Catalyzed by enzymes such as transacylases and transferases.
Major Products Formed
Acetyl-CoA: A key intermediate in the citric acid cycle.
Enoyl-CoA: An intermediate in the beta-oxidation pathway.
Various fatty acid derivatives: Formed through transesterification reactions.
Scientific Research Applications
Coenzyme A,S-octadecanoate has numerous applications in scientific research:
Chemistry: Used as a model compound to study enzyme kinetics and mechanisms of acyl transfer reactions.
Biology: Essential for studying metabolic pathways involving fatty acid metabolism and energy production.
Medicine: Investigated for its role in metabolic disorders and potential therapeutic applications in conditions such as obesity and diabetes.
Industry: Utilized in the production of biofuels and biodegradable plastics through microbial fermentation processes
Mechanism of Action
Coenzyme A,S-octadecanoate exerts its effects by acting as a carrier of acyl groups within cells. It forms thioester bonds with fatty acids, facilitating their transport and subsequent reactions in metabolic pathways. The molecular targets include enzymes involved in fatty acid oxidation, such as acyl-CoA dehydrogenase and enoyl-CoA hydratase. The pathways involved include the beta-oxidation pathway and the citric acid cycle, where it contributes to the generation of acetyl-CoA and energy production .
Comparison with Similar Compounds
Similar Compounds
Acetyl-CoA: Involved in the citric acid cycle and fatty acid synthesis.
Succinyl-CoA: Participates in the citric acid cycle and heme synthesis.
Malonyl-CoA: A key intermediate in fatty acid biosynthesis
Uniqueness
Coenzyme A,S-octadecanoate is unique due to its specific role in the metabolism of long-chain fatty acids. Unlike acetyl-CoA and succinyl-CoA, which are involved in broader metabolic processes, this compound is specifically tailored for the activation and transport of octadecanoic acid. This specificity makes it a valuable compound for studying long-chain fatty acid metabolism and its associated pathways .
Properties
Molecular Formula |
C39H70N7O17P3S |
---|---|
Molecular Weight |
1034.0 g/mol |
IUPAC Name |
S-[2-[3-[[4-[[[(2R,3S,4R,5R)-5-(6-aminopurin-9-yl)-4-hydroxy-3-phosphonooxyoxolan-2-yl]methoxy-hydroxyphosphoryl]oxy-hydroxyphosphoryl]oxy-2-hydroxy-3,3-dimethylbutanoyl]amino]propanoylamino]ethyl] octadecanethioate |
InChI |
InChI=1S/C39H70N7O17P3S/c1-4-5-6-7-8-9-10-11-12-13-14-15-16-17-18-19-30(48)67-23-22-41-29(47)20-21-42-37(51)34(50)39(2,3)25-60-66(57,58)63-65(55,56)59-24-28-33(62-64(52,53)54)32(49)38(61-28)46-27-45-31-35(40)43-26-44-36(31)46/h26-28,32-34,38,49-50H,4-25H2,1-3H3,(H,41,47)(H,42,51)(H,55,56)(H,57,58)(H2,40,43,44)(H2,52,53,54)/t28-,32-,33-,34?,38-/m1/s1 |
InChI Key |
SIARJEKBADXQJG-KBEKLFCESA-N |
Isomeric SMILES |
CCCCCCCCCCCCCCCCCC(=O)SCCNC(=O)CCNC(=O)C(C(C)(C)COP(=O)(O)OP(=O)(O)OC[C@@H]1[C@H]([C@H]([C@@H](O1)N2C=NC3=C(N=CN=C32)N)O)OP(=O)(O)O)O |
Canonical SMILES |
CCCCCCCCCCCCCCCCCC(=O)SCCNC(=O)CCNC(=O)C(C(C)(C)COP(=O)(O)OP(=O)(O)OCC1C(C(C(O1)N2C=NC3=C(N=CN=C32)N)O)OP(=O)(O)O)O |
Origin of Product |
United States |
Disclaimer and Information on In-Vitro Research Products
Please be aware that all articles and product information presented on BenchChem are intended solely for informational purposes. The products available for purchase on BenchChem are specifically designed for in-vitro studies, which are conducted outside of living organisms. In-vitro studies, derived from the Latin term "in glass," involve experiments performed in controlled laboratory settings using cells or tissues. It is important to note that these products are not categorized as medicines or drugs, and they have not received approval from the FDA for the prevention, treatment, or cure of any medical condition, ailment, or disease. We must emphasize that any form of bodily introduction of these products into humans or animals is strictly prohibited by law. It is essential to adhere to these guidelines to ensure compliance with legal and ethical standards in research and experimentation.